Irene Cara e Michele Autelli
Definition

Marburg hemorrhagic fever is a rare, severe type of hemorrhagic fever, which affects both humans and non-human primates. It is caused by the so called “Marburg virus” (Marburg is the city where it was first recognized): a genetically unique zoonotic RNA virus belonging to the Filovirus family, along with the five species of Ebola, the causative agents of a different type of hemorrhagic fever. Though caused by different viruses, the two diseases are clinically similar: both of them are rare and have the capacity to cause dramatic outbreaks with high fatality rates (up to 88%).
(Cdc Marburg Hemorrhagic fever)
(WHO Marburg Hemorrhagic fever)
Epidemiology
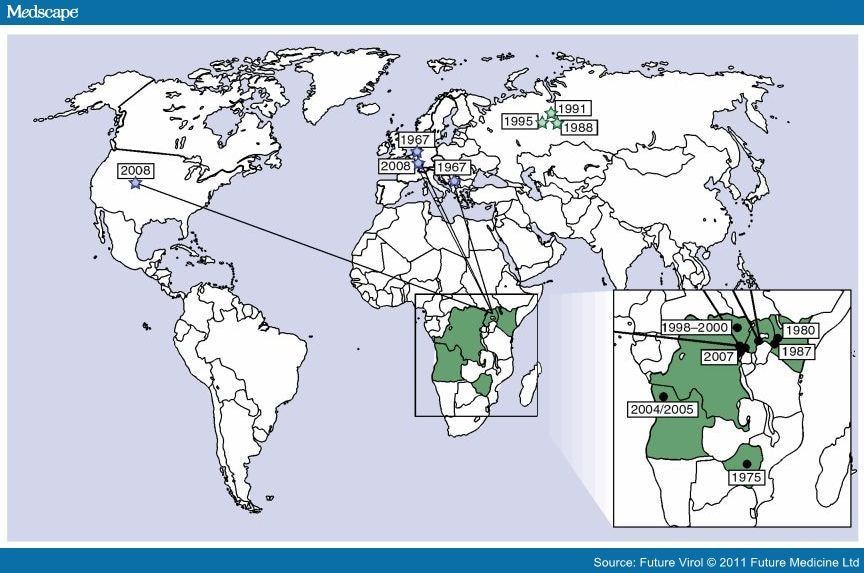
Marburg virus was first recognized in 1967, when outbreaks of hemorrhagic fever occurred simultaneously in laboratories in Marburg, Frankfurt and Belgrade. A total of 31 people became ill, including laboratory workers, as well as several medical personnel and family members who had cared for them. There were 7 deaths among the reported cases. The first people infected had been exposed to African green monkeys or their tissues. After this initial outbreak, the virus disappeared. It did not reemerge until 1975, when a traveler, most likely exposed in Zimbabwe, became ill in Johannesburg, South Africa. Confirmed cases of Marburg Hemorrhagic Fever (Marburg HF) have been reported in Uganda, Zimbabwe, the Democratic Republic of the Congo, Kenya, and Angola since that time. Cases of Marburg HF have also occurred outside Africa, though infrequently. In 2008, two independent cases were reported in travelers coming back from Uganda. Human infection results from prolonged exposure to mines or caves inhabited by Rousettus bats colonies. Just how the animal host first transmits Marburg virus to humans is unknown. However, humans who become ill with Marburg hemorrhagic fever may spread the virus to other people. Transmission is mainly human-to-human, resulting from close contact with the blood, secretions, organs or other bodily fluids of infected people.
(Cdc Marburg Hemorrhagic fever)
(WHO Marburg Hemorrhagic fever)
Pathogenesis

The severe illness results from a complex of pathogenetic mechanisms that enable the virus to suppress innate and adaptive immune responses (How Ebola and Marburg viruses battle the immune system, 2007 , Figure 3), infect and kill a broad variety of cell types, and elicit strong inflammatory responses and disseminated intravascular coagulation, producing a syndrome resembling septic shock.
(Pathogenesis of filoviral haemorrhagic fevers. 2004)
Risk factors
Because of the disease’s rarity and lethality, risk factors for transmission of Marburg virus have not been extensively investigated. Recent scientific studies implicate the African fruit bat, a sighted cave-dwelling bat widely distributed across Africa, as the reservoir host of the Marburg virus. Fruit bats infected with Marburg virus do not show obvious signs of illness. As we said before, it is still unknown how the virus is transmitted to humans, but a French cross-sectional study carried out in Democratic Republic of the Congo underlined a relation between the amount of time spent by miners in the caves and the probability of resulting positive to Marburg virus antibodies. Spread of the virus between humans has occurred in a setting of close contact, often in a hospital. Droplets of body fluids, or direct contact with persons, equipment, or other objects contaminated with infectious blood or tissues are all highly suspect as sources of disease. Burial ceremonies, where mourners have direct contact with the body of the deceased, can play a significant role in the transmission of Marburg, but it can also occur via infected semen. Transmission to health-care workers has been reported while treating Marburg patients, through close contact without the use of correct infection control precautions (contaminated injection equipment or through needle-stick injuries) and it is associated with more severe disease, rapid deterioration, and, possibly, a higher fatality rate.
(Cdc Marburg Hemorrhagic fever)
(WHO Marburg Hemorrhagic fever)
Symptoms
After an incubation period of 5-10 days, the onset of the disease is sudden and is marked by fever, chills, headache, and myalgia. Death occurs due to multiple organ dysfunction syndrome, fluid redistribution, hypotension, disseminated intravascular coagulation, and focal tissue necroses. The disease’s progression can be divided into 3 phases:
• Generalization Phase: Day 1 up to Day 5 from onset of clinical symptoms. MHF presents with a high fever (~40˚C) and a sudden, severe headache, with accompanying chills, fatigue, nausea, vomiting, diarrhea, pharyngitis, maculopapular rash, abdominal pain, conjunctivitis and malaise.
• Early Organ Phase: Day 5 up to Day 13. Symptoms include prostration, dyspnea, edema, conjunctival injection, viral exanthema, and CNS symptoms, including encephalitis, confusion, delirium, apathy, and aggression. Hemorrhagic symptoms typically occur late and herald the end of the early organ phase, leading either to eventual recovery or worsening and death. Symptoms include bloody stools, ecchymoses, blood leakage from venipuncture sites, mucosal or visceral hemorrhaging and possibly hematemesis.
• Late Organ Phase: Day 13 up to Day 21. Symptoms bifurcate into two constellations for survivors and fatal cases. Survivors will enter a convalescence phase, experiencing myalgia, fibromyalgia, hepatitis, asthenia, ocular symptoms and psychosis. Fatal cases continue to deteriorate, experiencing continued fever, obtundation, coma, convulsions, diffuse coagulopathy, metabolic disturbances, shock and death (tipically occurring between 8and 16 days from the onset).
(Marburg virus disease)
Complications
Recovery from Marburg hemorrhagic fever may be prolonged and accompanied by orchitis, recurrent hepatitis, transverse myelitis or uveitis. Other possible complications include inflammation of the testis, spinal cord, eye, parotid gland, or by prolonged hepatitis.
Virology

Genome
Like all mononegaviruses, Marburg virions contain non-infectious, linear non segmented, single-stranded RNA genomes of negative polarity that possesses inverse-complementary 3' and 5' termini, do not possess a 5' cap, are not polyadenylated, and are not covalently linked to a protein. Marburgvirus genomes are approximately 19 kb long and contain seven genes.
Structure
Like all filoviruses, marburgvirions are filamentous particles that may appear in the shape of a shepherd's crook or in the shape of a "U" or a "6", and they may be coiled, toroid, or branched. Marburgvirions are generally 80 nm in width, but vary somewhat in length. In general, the median particle length of marburgviruses ranges from 795–828 nm (in contrast to Ebola virions, whose median particle length was measured to be 974–1,086 nm ), but particles as long as 14,000 nm have been detected in tissue culture. Marburgvirions consist of seven structural proteins. At the center is the helical ribonucleocapsid, which consists of the genomic RNA wrapped around a polymer of nucleoproteins (NP). Associated with the ribonucleoprotein is the RNA-dependent RNA polymerase (L) with the polymerase cofactor (VP35) and a transcription activator (VP30). The ribonucleoprotein is embedded in a matrix, formed by the major (VP40) and minor (VP24) matrix proteins. These particles are surrounded by a lipid membrane derived from the host cell membrane. The membrane anchors a glycoprotein (GP1,2) that projects 7 to 10 nm spikes away from its surface. While nearly identical to ebolavirions in structure, marburgvirions are antigenically distinct.
Entry
Niemann–Pick C1 (NPC1) appears to be essential for Ebola and Marburg virus infection. NPC1 was shown to be critical to filovirus entry because it mediates infection by binding directly to the viral envelope glycoprotein. A later study confirmed the findings that NPC1 is a critical filovirus receptor that mediates infection by binding directly to the viral envelope glycoprotein and that the second lysosomal domain of NPC1 mediates this binding. This might imply that genetic mutations in the NPC1 gene in humans could make some people resistant to two of the deadliest known viruses affecting humans. Moreover, NPC1 may be potential therapeutic target for an Ebola and Marburg viruses anti-viral drug.
Replication
The marburg virus life cycle begins with virion attachment to specific cell-surface receptors, followed by fusion of the virion envelope with cellular membranes and the concomitant release of the virus nucleocapsid into the cytosol. The virus RdRp partially uncoats the nucleocapsid and transcribes the genes into positive-stranded mRNAs, which are then translated into structural and nonstructural proteins. Marburgvirus L binds to a single promoter located at the 3' end of the genome. Transcription either terminates after a gene or continues to the next gene downstream. This means that genes close to the 3' end of the genome are transcribed in the greatest abundance, whereas those toward the 5' end are least likely to be transcribed. The gene order is therefore a simple but effective form of transcriptional regulation. The most abundant protein produced is the nucleoprotein, whose concentration in the cell determines when L switches from gene transcription to genome replication. Replication results in full-length, positive-stranded antigenomes that are in turn transcribed into negative-stranded virus progeny genome copies. Newly synthesized structural proteins and genomes self-assemble and accumulate near the inside of the cell membrane. Virions bud off from the cell, gaining their envelopes from the cellular membrane they bud from. The mature progeny particles then infect other cells to repeat the cycle.
(Marburg virus)
Diagnosis
Marburg virus disease is clinically indistinguishable from Ebola virus disease and can also be confused with several Equatorial deseases such as other viral hemorrhagic fevers, falciparum malaria, typhoid fever, shigellosis, rickettsial diseases such as typhus, cholera, gram-negative septicemia, borreliosis such as relapsing fever or EHEC enteritis. Differential diagnosis can be achieved thanks to the analysis of patient medical history (exposure to wild life and places he recently visited). MVD can then be confirmed by isolation of marburgviruses or by detection of marburgvirus antigen or genomic or subgenomic RNAs in patient blood sample during the acute phase of MVD. Marburgvirus isolation is usually performed by inoculation of grivet kidney epithelial Vero E6 or MA-104 cell cultures or by inoculation of human adrenal carcinoma SW-13 cells, all of which react to infection with characteristic cytopathic effects. Filovirions can easily be visualized and identified in cell culture by electron microscopy due to their unique filamentous shapes, but electron microscopy cannot differentiate the various filoviruses alone despite some overall length differences.Immunofluorescence assays are used to confirm marburgvirus presence in cell cultures. Tests on clinical samples present an extreme biohazard risk and are conducted only under maximum biological containment conditions.
(Marburg virus disease)
Therapy
Because of the lack of effective therapy for Marburg virus disease, prevention plays a key role in term of avoiding further infections and deaths in humans. First of all, population must be aware of risk factors, so public health educational messages should focus on:
• Reducing the risk of bat-to-human transmission arising from prolonged exposure to mines or caves inhabited by fruit bats colonies, by subsidizing the use of gloves and masks.
• Reducing the risk of human-to-human transmission in the community arising from direct or close contact with infected patients, particularly with their body fluids.
• Reducing the risk of infection during the burial of the dead, that should be carried out in safety conditions.
In addition, prevention must be extended to health-care settings, where the risks for personnel is even higher: Marburg virus transmission associated with provision of health care has been reported when appropriate infection control measures have not been observed. Suspected or confirmed cases of Marburg Virus Disease must be approached with specific control measures and reinforcement of standard precautions, particularly hand hygiene, use of personal protective equipment (PPE) and safe injection practices. Laboratory workers are also at risk. Samples taken from suspected human and animal Marburg cases for diagnosis should be handled by trained staff and processed in suitably equipped laboratories.
In case of infection,treatment is primarily supportive in nature and includes minimizing invasive procedures, balancing fluids and electrolytes to counter dehydration, administering anticoagulants early in infection in order to prevent or control disseminated intravascular coagulation, administering pro-coagulants late in infection to control hemorrhaging, maintaining oxygen levels, pain management, and administering antibiotics or anti-mycotics to treat secondary infections.
(Marburg virus disease)