Gliotransmission
Gliotransmitters are chemicals released from glial cells, whose function is to facilitate neuronal communication between neurons and other glial cells. These substances are produced by several types of glial cells, but astrocytes are the ones playing the greatest role. The mechanisms on which this type of nervous communication is based are usually linked with Ca2+ signaling.
Glial cells were considered for a long time just as a type of support cells, whose primary function was to help to mantain homeostasis in brain and other parts of nervous system: in other words, they allow neurons to work in a steady, controlled, nutrient rich environment, in order for them to operate at the best of their possibilities. The name “neuroglia” comes from the Greek, where “glia” means “glue”: it therefore underlines the important role in ensuring stability in the CNS.

Astrocytes have been considered as the main type of glial cell due to their important function in nervous tissue homeostasis; they play a relevant role in a number of processes, such as mantaining the blood-brain barrier, storing glycogen as a source of energy to fuel neurons, metabolic support by producing lactate from glucose, vasomodulation of brain blood flow, repairing of lesion through the formation of a glial scar tissue, regulation of ions concentration in the extracellular space, synaptic homeostasis (e.g. uptaking the glutamate released by neurons, converting it into glutamine and provide this aminoacid back to the neuron).

Despite those functions, a role in nervous transmission for glial cells was not taken into account for a very long time, since they were seen just as passive, support elements. Glial cells cannot produce action potentials and therefore were not suspected as playing an important and active communicative role in the central nervous system, because synaptic transmission between neurons is initiated with an action potential. Anyway, recent studies have shown a number of possible functions in this field, thanks to the release of gliotransmitters, which now are thought to represent an important way to modulate the functioning of neurons, e.g. acting on neurotransmitters receptors to increase or decrease their activation, or even transmitting signals to neurons or other glial cells (Gliotransmission and the tripartite synapse, 2012).
How do astrocytes communicate?
Astrocytes are not merely supportive but are integrative, being able to receive inputs, assimilate information and send instructive chemical signals to other neighbouring cells including neurons. But which mechanisms lie under those functions?
At first, the excitatory neurotransmitter glutamate was found to be a major extracellular messenger that mediates these communications, because it can be released from astrocytes in a Ca2+ dependent manner and can stimulate extra-synaptic glutamate receptors in adjacent neurons, leading to a dynamic modification of synaptic transmission. However, recently other transmitters produced by glial cells, such as ATP, D-serine, taurine, neurosteroid or endozepines seem to play the greatest role.

Although glial cells are not excitable in terms of electrophysiology, they have been shown to generate synchronized Ca2+ transients (Ca2+ oscillations) through mechanisms of chemical coupling. Until recently, Ca2+ transients in astrocytes were thought to be totally dependent on neuronal activities, because astrocytes express a large variety of receptors for neurotransmitters and surround almost all synapses at which neurotransmitters are spilled over to stimulate astrocytes. In addition, however, astrocytes have been shown to release diffusible substances, the gliotransmitters, and Ca2+ transients in astrocytes are therefore also triggered by astrocytic activities, leading to propagation of Ca2+ transients or Ca2+ waves (Synchronization of Ca2+ oscillations: involvement of ATP release in astrocytes, 2010).

The activation of those Ca2+ waves can be induced, as it has been said before, by neurotransmitters which are released into the synaptic space surrounded by astrocytes terminations. Evidence of this mechanisms has been provided by research into the role of mGluR5 in astrocytes in the nucleus accumbens. This type of glutamate receptor has a major role in regulating Ca2+ signaling in astrocytes and, as a consequence, the Ca2+ dependent release of excitatory transmitters from these glia: activation of mGluR5 induces Ca2+ oscillations in NAcc astrocytes with the correlated appearance of NMDA receptor-dependent slow inward currents detected in nucleus accumbens neurons. In other words, glutamatergic afferents cause the sustained activation of astrocytes, which in turn excite the surrounding through extrasynaptic NMDA receptors: this might be involved in amplificating neuronal signals (mGluR5 stimulates gliotransmission in the nucleus accumbens, 2007).
The release of gliotransmitters can be mediated by mechanisms which are quite different from the classical ones. Some studies have shown the important role of connexin 43 (Cx43), a protein forming hemichannels, that is structures which can be described as the half of a gap junction channel. It has not been found out yet how this exactly works, anyway some gliotransmitters such as ATP or glutamate may be released from the astrocytes through those hemichannels rather than in the classical Ca2+ dependent way through vescicles: an increase in permeability might allow those chemicals to go out, through regulation by intracellular pathways. Some evidence has been provided by studies in which those emichannels were selectively inhibited by a specific peptide: many functions, which had been previously related to gliotransmission, appeared to be depressed, such as the fear memory consolidation in the basolateral amygdala, with amnesia for auditory fear conditioning in rats (Release of gliotransmitters through astroglial connexin 43 hemichannels is necessary for fear memory consolidation in the basolateral amygdala., 2012).

But what are those gliotransmitters? Among the several chemicals which are known to play an important role, we are now going to see four examples: D-serine, taurine, endozepines and ATP, even if many other substances may act this way (e.g. several neurosteroids are produced by glial cells and can act as gliotransmitters).
D-serine
The aminoacid D-serine is thought to have an extremely important role in memory, throught its action on NMDA receptors: notably, it interacts with the site which has been considered for a long time as the regulation site of glycine on the NMDA-R
The synthesis of D-serine require serine racemase, an enzyme which is found in astrocytes and whose role is to catalyze the formation of D-serine from L-serine, the form in which this amminoacid is normally contained in human proteins. The same enzyme, besides converting L-serine in its optical isomer, can be involved in the catabolism of this aminoacid, through elimination of water from L-serine, generating pyruvate and ammonia

D-serine is synthesized mainly in glial cells and it is released upon activation of glutamate receptors, therefore when the nearby synapsis is activated. Its concentration in the synaptic cleft controls the number of post-synaptic NMDA-R available for activation by glutamate, since for this receptor it is necessary the binding of both glutamate and D-serine (Regulation of N-methyl-D-aspartate receptors by astrocytic D-serine, 2009).

D-serine is therefore an endogenous co-agonist of NMDA-R, acting on the so-called “glycine site". Through this action, in many brain areas it governs the induction of long-term potentiation and long-term depression by regulating postsynaptic AMPA receptor density, which is mediated chiefly by NMDA-R activation. In this mechanism, serine is helped by other gliotransmitters: ATP and TNF-alpha trigger long-lasting increases in synaptic strength at glutamatergic hypothalamic and hippocampal inputs, respectively, through mechanisms that promote AMPA-R insertion (Glia: they make your memory stick!, 2007).

Long-term potentiation (LTP) of synaptic transmission represents the cellular basis of learning and memory: through these combined actions, therefore, astrocytes regulate synaptic transmission and plasticity and are extremely important for us to be able to store new memories.
The effects of D-serine on NMDA receptors have a relevant roles for other types of nervous function as well. This aminoacid can act by increasing the strenght of glutamatergic transmission in several synapses where glutamate acts on NMDA, or even by modulating the action of this transmitter on nearby glial cells.
Examples of this functions include:
- pain-induced behaviour: D-serine seems to have a major role in the development of emotional behaviour and response to noxious stimulation. Its role would be to favour, through an increase in NMDA receptor activity, the mechanism underlying neural plasticity in some areas such as amygdala. Changes in the strenght of synapses at this level would be involved in emotional changes brought about by chronic pain, as it has been seen in some research carried out on mice. The knock-out of the gene of serine racemase, the enzyme whose role is necessary to synthetize D-serine, involves a reduction in the NMDA-R mediated excitatory post-synaptic currents in amygdala (Functional roles of endogenous D-serine in the chronic pain-induced plasticity of NMDAR-mediated synaptic transmission in the central amygdala of mice, 2012). This also results in behavioural changes: while wild type mices show, when exposed to repeated noxious stimulation, an increase in the emission of ultrasonic vocalization and a decrease in locomotory activity, knock-out mice do not show any kind of adaptative change in behaviour (Functional roles of endogenous D-serine in pain-induced ultrasonic vocalization, 2012);
- neurovascular coupling: the release of D-serine seems to improve the mechanism by which astrocytes, stimulated by glutamate released by neurons, make the blood flow to a highly activated area increase. The vasodilation in arterioles providing blood to that area is mediated by several chemicals, among which one of the most important is nitric oxide. The release of NO produced by eNOS in a pathway involving astrocytes would rely on the activation of NMDA-R thanks to D-serine production: the dilatory responses is dramatically reduced by scavenging D-serine or by blocking the receptor (Astrocyte-induced cortical vasodilation is mediated by D-serine and endothelial nitric oxide synthase, 2012);
- retinal ganglion cells: in the retina, NMDA receptor seems to play an important role in ganglion cells activation; for this to happen, the D-serine release is needed. This would be triggered by AMPA-R activation, and the result might be important for a normal trasmission in ON-center response (AMPA receptor-dependent, light-evoked D-serine release acts on retinal ganglion cell NMDA receptors, 2012).
D-serine might be very important in nervous system pathology as well: a disruption in the normal balance between synthesis and elimination of this chemicals is thought to be one of the possible causes for disease such as schizophrenia, ALS or even Alzheimer disease. In many neurodegenerative conditions, D-serine levels seem to be changed, for reasons such as an increase in the induction of the serine racemase: that’s why inhibitors of this enzyme are thought to be going to play an important role in neurologic therapies (Inhibition of human serine racemase, an emerging target for medicinal chemistry, 2011).
In schizophrenia, an increase in the availability of D-serine seems to improve the symptoms of the disease: oral administration has been showed to have positive effect on patient. On the other hand, several findings have linked low levels of D-serine to schizophrenia: D-serine concentrations in serum and cerebrospinal fluid have been reported to be decreased in schizophrenic patients while human D-aminoacid oxidase activity and expression are increased.
The D-aminoacid oxidase, or DAAO, is one of the enzymes involved in D-serine catabolism: one pathway requires the same enzyme which syntethize it (the racemase), whereas DAAO provides an alternative way based on oxidative deamination. This enzyme is contained in the peroxysomes and belongs to the family of FAD-dependent oxidoreductases: it acts on the CH-NH2 group of D-amino acid donors with oxygen as acceptor.

Inhibitors of DAAO improve the conditions of patients affected by schizophrenia, suggesting that the disease might be treated by increasing the activity of NMDA-R through an action on this gliotransmitter (D-Amino Acid Oxidase Inhibitors as a Novel Class of Drugs for Schizophrenia Therapy, 2012).
The most relevant role for D-serine in pathology anyway seems to concern amyotrophic lateral sclerosis pathogenesis (ALS). ALS is a motor neuron disease in which spinal cord neuros are destroyed in a degenerative process: according to recent research, glial involvement seems to be essential for ALS-related motoneuronal death. This would have a relevant impact on our possibilities to understand the mechanism underlying the pathology, and obviously to develop new drugs with new targets to fight against this severe disease.
D-serine might produce a strong activation of NMDA receptors on motor neurons, and this would involve a relevant inward current of calcium ions into the neuron: the increase in the levels of calcium can activate in the cells several pathway leading to neuronal death. It would be therefore a typical mechanism of excitotoxicity, strongly influenced by the levels of D-serine (D-serine is a key determinant of glutamate toxicity in amyotrophic lateral sclerosis, 2007).

This hypothesis has been proved by many observations:
- familial forms of ALS are associated with mutations of the DAAO gene, whose absence reduce the elimination rate of D-serine bringing about accumulation of this gliotransmitter in the spinal cord (Familial amyotrophic lateral sclerosis is associated with a mutation in D-amino acid oxidase, 2010);
- a reduced activity of DAAO is involved in several forms of ALS (D-amino acid oxidase controls motoneuron degeneration through D-serine, 2012);
- serine racemase, the enzyme producing D-serine, is increased in cell stress and inflammatory processes, which are thought to contribute to the onset of sporadic ALS and in disease progression (The role of D-amino acids in amyotrophic lateral sclerosis pathogenesis: a review, 2012);
- mice in which serine racemase is not functioning have earlier symptom onset, yet show a slower progression of the disease (Paradoxical roles of serine racemase and D-serine in the G93A mSOD1 mouse model of amyotrophic lateral sclerosis, 2012).
Taurine
Taurine is the 2-aminoethanesulfonic acid, that is a sulfonic acid containing an amino group.
It is an organic osmolyte involved in cell volume regulation, and provides a substrate for the formation of bile salts, with which it can be conjugated. It plays a role in the modulation of intracellular free calcium concentration, and although it is not incorporated into proteins, taurine is one of the most abundant amino acids in the brain, retina, muscle tissue, and organs throughout the body.
The synthesis of taurine results from cysteine degradation; those reactions take place in astrocytes, and this seems to provide evidence for a role of taurine in gliotransmission and CNS functions; some studies show that neurons are necessary as well to complete the synthesis, in a metabolic cooperation between those two types of cells.
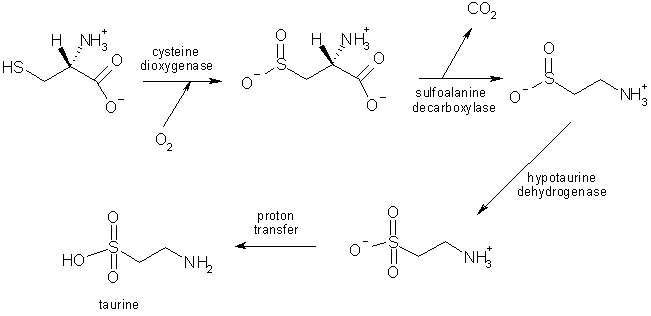
Taurine is considered to take part in several nervous processes:
- mood: taurine is a common ingredient in energy drinks (e.g. Red Bull), because it is believed to have a cognition- or mood-boosting function.

These effects might be due to the action of taurine on strychnine-sensitive glycine receptors (Gly-Rs): this seems to have an anti-anxiety effect and can affect the functioning of nucleus accumbens. Taurine could act on nucleus accumbens Gly-Rs activating a loop involving endogenous activation of nicotinic acetylcholine receptors (nAChR) in the VTA (ventral tegmental area): this area contains dopaminergic neurons projecting to the accumbens, involved in reward-related behaviours and addictive processes, such as alcoholism and drug addiction. Therefore, taurine would have a positive effect on mood, acting in a way which is similar to the mechanism used by ethanol; this could be used to develop drugs to treat alcoholism and alcohol withdrawal, such as acamprosate (Taurine elevates dopamine levels in the rat nucleus accumbens; antagonism by strychnine, 2006);

- neuroprotection: taurine reduces oxydative stress in neurons, and dysregulation of the taurine transporter in high-glucose-exposed human Schwann cells might implications for pathogenesis of diabetic neuropathy. Taurine might prevent neuronal death by blocking apoptotic pathways mediated by calpain and caspase-3, caspase-8 and caspase-9 (Taurine reduces nitrosative stress and nitric oxide synthase expression in high glucose-exposed human Schwann cells, 2012);
- modulation of GABA-A receptors: taurine-fed mice show changes in the GABaergic system, such as the subunit composition of this receptor, which makes them react differently to drugs affecting the functioning of this neurotransmitters, probably with a protective effect on seizures; moreover, this mechanism has been proposed to improve cognitive implications in ageing, by reducing the naturally occurring age-related decline in cognitive functions (Pharmacological characterization of GABAA receptors in taurine-fed mice, 2010);
- modulation of osmosensitive neurons: astrocytes can release taurine through volume-regulated anion channels (VRACs), and it has been hypothesized that taurine released from glial cells is capable of inhibiting action potential firing by activating neuronal GlyRs in hypothalamic supraoptic nucleus (SON), which is involved in osmolarity control through the secretion of vasopressin. Taurine is released in conditions of hyponatremia, due to cell swelling, and it suppresses therefore vasopressin to reduce water reabsorption in the kidneys (Taurine release by astrocytes modulates osmosensitive glycine receptor tone and excitability in the adult supraoptic nucleus, 2012).
Endozepines
Endozepines are endogenous substances which are able to modulate the functioning of GABA-A receptors. The name comes from the fact that they had been discovered after the development of benzodiazepines such as Diazepam: those drugs act on a site of the receptors by modulating sensitivity to gamma-aminobutyric acid increasing GABAergic transmission, and this structure must have been developed through evolution in order for some kind of endogenous chemical to act.

The diazepam binding inhibitor (DBI) was a peptide originally isolated from the brain as an intrinsic ligand of the benzodiazepine binding site on the GABA-A. Its wide-spread distribution in non-neural cells, outside the brain as well, suggests that DBI has various functions other than GABA-mediated neurotransmission: notably, several studies have revealed a definite existence of DBI in non-neuronal supporting cells in both the central and peripheral nervous systems (Cellular localization of the diazepam binding inhibitor in glial cells with special reference to its coexistence with brain-type fatty acid binding protein, 2002).
The role of DBI in neuroglial cells have not been clearly established yet, but there are many hypotheses:
- hypothalamic glucose sensing: DBI and its processing product the octadecaneuropeptide (ODN), collectively named endozepines, are secreted by astroglia, and ODN is a potent anorexigenic factor. Intracerebroventricular administration of glucose in rats increases DBI expression in hypothalamic glial-like tanycytes, and it may stimulate endozepine secretion from hypothalamic explants as well. These substances seem to act on melanocortin-3/4 receptors, which are known to be involved in the anorexigenic signaling of MSH: the raise of glucose blood levels after a meal contributes to trigger the secretion of this transmitter, which stops the need for eating. The anorexigenic effects of centrally injected glucose or ODN agonist were suppressed by blockade of the M3/4 receptors, suggesting that glucose-sensing involves endozepinergic control of the melanocortin pathway (Gliotransmission and Brain Glucose-Sensing: Critical Role of Endozepines., 2012).

According to these hypothesis, glial cells, through the production of endozepines, may relay peripheral signals to neurons involved in the central regulation of energy homeostasis: another piece of evidence is the fact that acute fasting dramatically reduces DBI mRNA levels in the hypothalamus and the ependyma bordering the third and lateral ventricles (Acute food deprivation reduces expression of diazepam-binding inhibitor, the precursor of the anorexigenic octadecaneuropeptide ODN, in mouse glial cells. , 2010);
- neuroprotection: ODN has been shown to stimulate the activity of enzymes such as superoxide dismutases (SOD) and catalase, preventing oxidative stress-induced apoptotic cell death. Hydrogen peroxide can induce apoptotic death in rat astrocytes by activating pathways responding to ROS signaling: if astrocytes are pre-treated with ODN, ROS accumulation is reduced and this results in a better survival, and this seem to be due to an increased activity of scavenger enzymes, since selective blockers of SOD and catalase suppress its positive effect (Protective effect of the octadecaneuropeptide on hydrogen peroxide-induced oxidative stress and cell death in cultured rat astrocytes., 2011);
- neurosteroids action: endozepines and neurosteroids can both act on the same receptor, GABA-A, by increasing in different ways the strenght of GABAergic transmission. These two types of chemicals seem to work together, each by reinforcing the synthesis of the other. ODN stimulates the biosynthesis of neurosteroids through activation of central-type benzodiazepines receptors, as it has been seen in frog hypothalamic glial cells; on the other hand, neurosteroid can trigger endozepines secretion. DBI can be obtained by proteolitic cleavage of acyl-CoA binding protein (ACBP), which is released and cleaved to the active neuropeptides by protease activity on the surface of astrocytes: chemicals such as pregnenolone seem to trigger that mechanism. The effects of steroids in neurons will be reinforced by the release of endozepines from astrocytes, strenghtening the effects of GABAergic transmission, e.g. in controlling neurons to prevent seizures (The octadecaneuropeptide ODN stimulates neurosteroid biosynthesis through activation of central-type benzodiazepine receptors, 2001; Pregnenolone sulfate and cortisol induce secretion of acyl-CoA-binding protein and its conversion into endozepines from astrocytes, 2010).
ATP
ATP as a number of functions as a gliotransmitter. It is released by astrocites with mechanisms which have not been clearly understood yet: some may be calcium-dependent, whereas others might involve release through astrocyte connexin 43 hemichannels after permeabilization triggered e.g. by hypoxia.
Astrocytes express various neurotransmitter receptors including P2 receptors, release ATP in response to various stimuli and respond to extracellular ATP to cause various physiological responses. The intercellular communication "Ca2+ wave" in astrocytes was found to be mainly mediated by the release of ATP and the activation of P2 receptors, suggesting that ATP is a dominant gliotransmitter between astrocytes.
Ca2+ transients in astrocytes are triggered not only by neuronal activity, but also by astrocytic activities, leading to propagation of Ca2+ waves. In these processes, the gliotransmitter ATP and activation of P2Y receptors play central roles, with a prominent role for the P2Y1 receptor subtype. Interestingly, astrocytes evoke Ca2+ transients even when neurons are not present, suggesting that astrocytes themselves can initiate and control Ca2+ transients, probably through ATP release (Regulation of cell-to-cell communication mediated by astrocytic ATP in the CNS, 2005).
The main function for neuroglial ATP seems to be heterosynaptic suppression: in hyppocampus ATP and adenosine, one of its degradation products, can contribute to “switch off” glutamatergic transmission when released by astrocytes, by decreasing presynaptic exocytotic events. Glutamate release accompanying neuronal activity in the glutamatergic synapsis also activates non-NMDA receptors of nearby astrocytes: this makes Ca2+ levels in the glial cells increase, and triggers ATP release from these cells. ATP in turn tonically suppresses glutamatergic synapses via presynaptic P2Y receptors: the presynaptic neuron, in other words, decreases the amount of glutamate released. This is therefore a kind of activity-dependent down-regulation of synaptic transmission via presynaptic mechanisms (ATP released by astrocytes mediates glutamatergic activity-dependent heterosynaptic suppression, 2003).
In astrocytes ATP also seems to provide control over other types of glial cells, above all injury responses, with a prominent role for the P2X7 receptor subtype; ATP might control microglial behaviour and regulate oligodendrocyte differentiation and myelination (ATP: a ubiquitous gliotransmitter integrating neuron-glial networks, 2011).
