Introduction

One of the most peculiar elements in ancient Norse sagas were tales involving a specialized group of Viking warriors known as the berserkers or berserks. Unlike the regular fighters, these attackers wore no armour, but plunged into battle dressed in the skins of wolves or bears, taking advantage of the natural human fear of wild beasts. The berserks fought so fiercely, it was believed they were actually able to shape-shift and turn into wild predators.
In preparation for battle, the berserkers entered a state of near-madness which endowed them with superhuman strength. The condition began with shivering and chills, followed by their faces swelling and changing colour. They were then seized by fits of anger which grew until they erupted into a lengthy period of uncontrollable rage. The berserkers would scream and howl like wild animals and gnaw mindlessly on the edge of their shields. As the battle began, they advanced wildly, seeming to lose all human reason and cut down everything in their path, friend or foe. They were so strong, no one or nothing could withstand their assault. In this state of frenzy, they were oblivious to pain. Even though they were mortally wounded, they continued fighting, thus further terrifying an opponent.
This fury, which was called berserkergang, occurred not only in the heat of battle, but also during laborious work.
There was only one way to overcome the berserkers: following each episode of frenzy, they were left in state of extreme feebleness, which lasted from one to several days.

In 1015 A.D., King Erik outlawed berserkers. By the 12th century, organised berserker war-bands had disappeared.
Unintentionally, the berserkers left an interesting legacy in a common expression, going berserk, used colloquially to describe a person who is acting in a wild rage or in an uncontrolled and irrational manner. Furthermore, the word berserker today applies to anyone who fights with reckless abandon and disregard to even his own life, a concept used during the Vietnam War and in Vietnam-inspired literature.
Modern scholars have offered different theories to explain the berserkers' bouts of frenzy: the consumption of hallucinatory plants, drinking massive quantities of alcohol, epilepsy, mental illness or genetic flaws. Nevertheless this theories are not entirely satisfactory, because they aren't applicable at all related cases we read about from literature - for instance, at Vietnam warfighters.
Whatever the cause, it is much more interesting to analyze how the human body is so capable of adapt physiologically also to a so unnatural and stressful state as the extreme fury of a battle.
"Going berserk"
Specific behavioral patterns due to hypothalamus and amygdala
"Punishment centers" have been found in the central gray area surrounding the aqueduct of Sylvius in the mesencephalon and extending upward into the periventricular zone of the hypothalamus . An emotional pattern that involves these and other limbic structures, and has been well characterized, is the rage pattern, described as follows.

Strong stimulation of the punishment centers of the brain, especially in the periventricular zone of the hypothalamus and in the lateral hypothalamus, causes the animal to (1) develop a defense posture, (2) extend its claws, (3) lift its tail, (4) hiss, (5) spit, (6) growl, and (7) develop piloerection, wide-open eyes, and dilated pupils. Furthermore, even the slightest provocation causes an immediate savage attack. This is approximately the behavior that one would expect from an animal being severely punished, and it is a pattern of behavior that is called rage.
In general, the amygdala is also important to the coordinated responses to stress and integrates many behavioral reactions involved in the survival of the individual or of the species, particularly to stress and anxiety. The stimulation in the amygdala can cause almost all the same effects as those elicited by direct stimulation of the hypothalamus, plus other effects.
Effects initiated from the amygdala and then sent through the hypothalamus include (1) increases or decreases in arterial pressure, (2) increases or decreases in heart rate, (3) increases or decreases in gastrointestinal motility and secretion, (4) defecation or micturition, (5) pupillary dilation or, rarely, constriction, (6) piloerection, and (7) secretion of various anterior pituitary hormones, especially the gonadotropins and adrenocorticotropic hormone ( see below in "Role of cortisol").
In addition, stimulation of certain amygdaloid nuclei can cause a pattern of rage, escape, punishment and fear similar to the rage pattern elicited from the hypothalamus, as described earlier.
In summary, both data and theory have supported the notion that the hypothalamus is integral to rage production. The septum and amygdala are both extensively interconnected with the hypothalamus, thus providing neuroanatomical support for a hypothalamic role in rage. Indeed, lesion studies with cats and case studies with humans support the role of the hypothalamus acts to balance the septum and amygdaloid regions to promote normal levels of hostility and that prefrontal and temporal regions also interact, via the inhibitory uncinate tract, to yield stable aggressions levels
It seems therefore likely that, when one of this "set points" is inhibited or become ineffective, for one reason or another, it can initiate a chain of neuroanatomical events that produce their own cognitive, emotional and behavioral features: when summated, these features equate to rage.
Control of the autonomic nervous system
Central control of the autonomic nervous system (ANS) outputs involves several interconnected areas distributed throughout the neuraxis. This central autonomic network has a critical role in moment-to-moment control of visceral function, homeostasis and adaptation to internal or external challenges. The functions of the central autonomic network are organized in four hierarchical levels that are closely interconnected: spinal, bulbopontine, pontomesencephalic and forebrain levels. The spinal level mediates segmental sympathetic or sacral parasympathetic reflexes and is engaged in a stimulus-specific patterned responses under the influence of the other levels. The bulbopontine (lower brainstem) level is involved in reflex control of circulation, respiration, gastrointestinal function and mictrurition. The pontomesencephalic (upper brainstem, midbrain) level integrates autonomic control with pain modulation and integrated behavioral responses to stress. The forebrain level includes the hypothalamus, which is involved in integrated control of autonomic and endocrine responses for homeostasis and adaptation, and components of the anterior limbic circuit, including the insula, anterior cingulate cortex, and amygdala, which are involved in integration of bodily sensation with emotional and goal-related autonomic responses.

The routes by which the hypothalamus and other forebrain structures influence the visceral and somatic motor systems are complex. The major targets of the hypothalamus lie in the reticular formation, the tangled web of nerve cells and fibers in the core of the brainstem. This structure contains over 100 identifiable cell groups. The reticular neurons receive hypothalamic input from and feed into both somatic and autonomic effector systems in the brainstem and spinal cord. Their activity can therefore produce widespread visceral motor and somatic motor responses, often overriding reflex function and sometimes involving almost every organ in the body.
Thus, in a state of rage, as in our instance of the berzerkers, which is elicited to a great extent by stimulating the hypothalamus, signals are transmitted downward through the reticular formation of the brain stem and into the spinal cord to cause massive sympathetic discharge; most of the "sympathetic events" described below ensue immediately. This is the renowned "sympathetic alarm reaction" or fight or flight reaction . A human (as well as any mammalian) in this state decides almost instantly whether to stand and fight or to run; in either event, the sympathetic alarm reaction makes a soldier's subsequent activities vigorous.
Action of the sympathetic nervous system
Chromaffin cells, neuroendocrine cells found mostly in the medulla of the adrenal glands, are intimately connected with the orthosympathetic division of the autonomic nervous system. They are thus considered as modified postganglionic neurons, and preganglionic autonomic nerve fibers lead to them directly from the central nervous system.
Chromaffin cells release catecholamines , on the average about 80% of epinephrine (adrenaline) and 20% of norepinephrine (noradrenaline) into the circulating blood, and these two hormones in turn are carried in the blood to all tissues of the body (similarly to secretory neurons of the hypothalamus); they can also send paracrine signals.
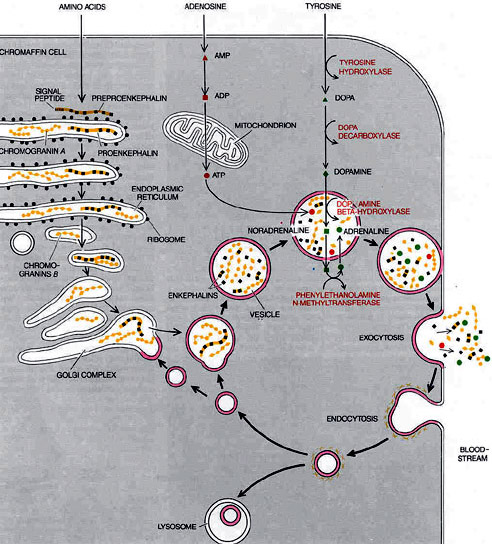
The circulating norepinephrine causes constriction of essentially all the blood vessels of the body; it also causes increased activity of the heart, inhibition of the gastrointestinal tract, dilation of the pupils of the eyes, and so forth. Epinephrine causes almost the same effects; it has 5 to 10 times as great a metabolic effect as norepinephrine.
Circulating epinephrine causes increased heart rate and inotropy (β 1 -adrenoreceptor mediated); vasoconstriction in most systemic arteries and veins (postjunctional α 1 and α 2 adrenoreceptors); vasodilation in muscle and liver vasculatures at low concentrations (β 2-adrenoceptor) but vasoconstriction at high concentrations (α 1-adrenoreceptor mediated).
The overall cardiovascular response to low-to-moderate circulating concentrations of epinephrine is increased cardiac output and a redistribution of the cardiac output to muscular and hepatic circulations with only a small change in mean arterial pressure. Although cardiac output is increased, arterial pressure does not change much because the systemic vascular resistance falls due to β 2-adrenoceptor activation.
At high plasma concentrations, epinephrine increases arterial pressure because of binding to α-adrenoceptors on blood vessels, which offsets the β 2-adrenoreceptor mediated vasodilation. The overall cardiovascular response is increased cardiac output and systemic vascular resistance, which results in an elevation in arterial blood pressure. Heart rate, although initially stimulated by norepinephrine, decreases due to activation of baroreceptors and vagal-mediated slowing of the heart rate.
Epinephrine and norepinephrine are almost always released by the adrenal medullae at the same time that the different organs are stimulated directly by generalized sympathetic activation. Therefore, the organs are actually stimulated in two ways: directly by the sympathetic nerves and indirectly by the adrenal medullary hormones. The two means of stimulation support each other, and either can, in most instances, substitute for the other, even though an important value of the adrenal medullae is the capability of epinephrine and norepinephrine to stimulate structures of the body that are not innervated by direct sympathetic fibers.
System discharge at the same time; this increases in many ways the ability of the body to perform vigorous muscle activity. In particular, we can appreciate increased arterial pressure; increased blood flow to active muscles, concurrent with decreased blood flow to organs such as the gastrointestinal tract and the kidneys, that are not needed for rapid motor activity; increased rates of cellular metabolism throughout the body; increased blood glucose concentration; increased glycolysis in the liver and in muscle; increased muscle strength; increased mental activity; increased rate of blood coagulation.

The sum of these effects permits a person to perform far more strenuous physical activity than would otherwise be possible. Because either mental or physical stress can excite the sympathetic system, it is frequently said that the purpose of the sympathetic system is to provide extra activation of the body in states of stress: this is called the sympathetic stress response.
Pain control
The degree to which a person reacts to pain varies tremendously. This results partly from a capability of the brain itself to suppress input of pain signals to the nervous system by activating a pain control system, called an analgesia system.
The analgesia system consists of three major components: (1) The periaqueductal gray and periventricular areas of the mesencephalon and upper pons surround the aqueduct of Sylvius and portions of the third and fourth ventricles. Neurons from these areas send signals to (2) the nucleus raphe magnus, a thin midline nucleus located in the lower pons and upper medulla, and the nucleus reticularis paragigantocellularis, located laterally in the medulla. From these nuclei, second-order signals are transmitted down the dorsolateral columns in the spinal cord to (3) a pain inhibitory complex located in the dorsal horns of the spinal cord. At this point, the analgesia signals can block the pain before it is relayed to the brain.
Several transmitter substances are involved in the analgesia system; especially involved are enkephalin and serotonin. Many nerve fibers derived from the periventricular nuclei and from the periaqueductal gray area secrete enkephalin at their endings. Thus, the endings of many fibers in the raphe magnus nucleus release enkephalin when stimulated.
Fibers originating in this area send signals to the dorsal horns of the spinal cord to secrete serotonin at their endings. The serotonin causes local cord neurons to secrete enkephalin as well. The enkephalin is believed to cause both presynaptic and postsynaptic inhibition of incoming type C and type Aδ pain fibers where they synapse in the dorsal horns. Thus, the analgesia system can block pain signals at the initial entry point to the spinal cord. In fact, it can also block many local cord reflexes that result from pain signals.
More than 40 years ago it was discovered that injection of minute quantities of morphine either into the periventricular nucleus around the third ventricle or into the periaqueductal gray area of the brain stem causes an extreme degree of analgesia. In subsequent studies, it has been found that morphine-like agents, mainly the opiates, also act at many other points in the analgesia system, including the dorsal horns of the spinal cord. Because most drugs that alter excitability of neurons do so by acting on synaptic receptors, it was assumed that the “morphine receptors” of the analgesia system must be receptors for some morphine-like neurotransmitter that is naturally secreted in the brain. Therefore, an extensive search was undertaken for the natural opiate of the brain.
About a dozen such opiate-like substances have now been found at different points of the nervous system; all are breakdown products of three large protein molecules: proopiomelanocortin , proenkephalin , and prodynorphin. Among the more important of these opiate-like substances are β-endorphin, met-enkephalin, leu-enkephalin and dynorphin. The two enkephalins are found in the brain stem and spinal cord, in the portions of the analgesia system described earlier, and β-endorphin is present in both the hypothalamus and the pituitary gland. Dynorphin is found mainly in the same areas as the enkephalins, but in much lower quantities.
Thus, although the fine details of the brain’s opiate system are not understood, activation of the analgesia system by nervous signals entering the periaqueductal gray and periventricular areas, or inactivation of pain pathways by morphine-like drugs, can almost totally suppress many pain signals entering through the peripheral nerves.
Role of cortisol
Cortisol , known more formally as hydrocortisone, is a steroid hormone, more specifically a glucocorticoid, produced by the zona fasciculata of the adrenal cortex.
It is released in response to stress and a low level of blood glucocorticoids. Its primary functions are to increase blood sugar through gluconeogenesis; suppress the immune system; and aid in fat, protein and carbohydrate metabolism. It also decreases bone formation. Various synthetic forms of cortisol are used to treat a variety of diseases.
Almost any type of physical or mental stress can lead within minutes to greatly enhanced secretion of ACTH and consequently cortisol as well, often increasing cortisol secretion as much as 20-fold. This effect was demonstrated by the rapid and strong adrenocortical secretory responses after trauma, demonstrated by an experiment in which corticosteroid formation and secretion increased sixfold in a rat within 4 to 20 minutes after fracture of two leg bones. Some of the different types of stress that increase cortisol release are: traumas of almost any type; infections; intense heat or cold; almost any debilitating disease.

Even though we know that cortisol secretion often increases greatly in stressful situations, we are not sure why this is of significant benefit to the animal. One possibility is that the glucocorticoids cause rapid mobilization of amino acids and fats from their cellular stores, making them immediately available both for energy and for synthesis of other compounds, including glucose, needed by the different tissues of the body. Indeed, it has been shown in a few instances that damaged tissues that are momentarily depleted of proteins can use the newly available amino acids to form new proteins that are essential to the lives of the cells. Also, the amino acids are perhaps used to synthesize other essential intracellular substances such as purines, pyrimidines, and creatine phosphate, which are necessary for maintenance of cellular life and reproduction of new cells. But all this is mainly supposition. It is supported only by the fact that cortisol usually does not mobilize the basic functional proteins of the cells, such as the muscle contractile proteins and the proteins of neurons, until almost all other proteins have been released. This preferential effect of cortisol in mobilizing labile proteins could make amino acids available to needy cells to synthesize substances essential to life.
When tissues are damaged by trauma, by infection with bacteria, or in other ways, they almost always become "inflamed". When large amounts of cortisol are secreted or injected into a person, the cortisol has two basic anti-inflammatory effects: it can block the early stages of the inflammation process before inflammation even begins, or, if inflammation has already begun, it causes rapid resolution of the inflammation and increased rapidity of healing. Cortisol has some effects in preventing inflammation; these are described as follows.
1. Cortisol stabilizes the lysosomal membranes. This is one of its most important anti-inflammatory effects, because it's much more difficult than normal for the membranes of intracellular lysosomes to rupture. Therefore, most of the proteolytic enzymes that are released by damaged cells to cause inflammation, which are mainly stored in the lysosomes, are released in greatly decreased quantity.
2. Cortisol decreases the permeability of the capillaries, probably as a secondary effect of the reduced release of proteolytic enzymes . This prevents loss of plasma into the tissues.
3. Cortisol decreases both migration of white blood cells into the inflamed area and phagocytosis of the damaged cells. These effects probably result from the fact that cortisol diminishes the formation of prostaglandins and leukotrienes that otherwise would increase vasodilation, capillary permeability, and mobility of white blood cells.
4. Cortisol suppresses the immune system, causing lymphocyte reproduction to decrease markedly. The T lymphocytes are especially suppressed. In turn, reduced amounts of T cells and antibodies in the inflamed area lessen the tissue reactions that would otherwise promote the inflammation process.
5. Cortisol attenuates fever mainly because it reduces the release of interleukin-1 from the white blood cells, which is one of the principal excitants to the hypothalamic temperature control system. The decreased temperature in turn reduces the degree of vasodilation.
Thus, cortisol has an almost global effect in reducing all aspects of the inflammatory process. How much of this results from the simple effect of cortisol in stabilizing lysosomal and cell membranes versus its effect to reduce the formation of prostaglandins and leukotrienes from arachidonic acid in damaged cell membranes and other effects of cortisol is unclear.
Even after inflammation has become well established, the administration of cortisol can often reduce inflammation within hours to a few days. The immediate effect is to block most of the factors that are promoting the inflammation. But in addition, the rate of healing is enhanced. This probably results from the same, mainly undefined, factors that allow the body to resist many other types of physical stress when large quantities of cortisol are secreted. Regardless of the precise mechanisms by which the anti-inflammatory effect occurs, this effect of cortisol plays a major role in combating certain types of diseases characterized by severe local inflammation, when the harmful effects on the body are caused mainly by the inflammation itself and not by other aspects of the disease. When cortisol or other glucocorticoids are administered to patients with these diseases, almost invariably the inflammation begins to subside within 24 hours. And even though the cortisol does not correct the basic disease condition, merely preventing the damaging effects of the inflammatory response, this alone can often be a lifesaving measure.
Conclusion
It is therefore plausible that a stressful environmental conditions or stimuli can activate part of the lymbic system. This activation can lead, as described, at a trance-like state of rage via hypothalamus/amygdala-sympathetic nervous system. Thanks to potent opiate-like effects of the enkephalins, in this state nothing can give rise to pain feelings, just as it is said to be happened to Berserkers, as described in Norse literature.
In this context, according to the sources of history and literature, we realize that cortisol played one of the leading roles in hypothetic berzerkers' recovery. In fact, most likely only a few berzerkers could enjoy the privilege of surviving the wounds: in addition to deadly blows received in battle (the most), infections from wounds and harsh climatic conditions often had the better of mighty Viking warriors. This is more or less true for all cases in history where such behavior have been observed.
References
Azami J., Llewelyn M.B., Roberts M.H., The contribution of nucleus reticularis paragigantocellularis and nucleus raphe magnus to the analgesia produced by systemically administered morphine, investigated with the microinjection technique.
Bard P., A diencephalic mechanism for the expression of the rage with special reference to the sympathetic nervous system
Dajas-Bailador F., Wonnacott S., Nicotinic acetylcholine receptors and the regulation of neuronal signalling.
Dampney R.A., Horiuchi J., Tagawa T., Fontes M.A., Potts P.D., Polson J.W., Medullary and supramedullary mechanisms regulating sympathetic vasomotor tone.
De Bosscher K., Vanden Berghe W., Haegeman G., The interplay between the glucocorticoid receptor and nuclear factor-kappaB or activator protein-1: molecular mechanisms for gene repression.
Goldstein D.S., Cathecolamines and stress.
Guyton A.C., Hall J.E., Textbook of medical physiology.
Heinricher M.M., Rosenfeld J.P., Microinjection of morphine into nucleus reticularis paragigantocellularis of the rat: suppression of noxious-evoked activity of nucleus raphe magnus neurons.
LeDoux J., The Emotional Brain, Fear, and the Amygdala Emotional Brain, Fear, and the Amygdala
McCoy D., Berserkers and other shamanic warriors.
Panin L.E., Maksimov V.F., Khoshchenko O.M., Korostyshevskaia I.M., Effect of combined glucocorticoids and low density lipoproteins on structural and functional changes in hepatocytes and Kupffer cells.
Purves D., Augustine G.J., Fitzpatrick D., Katz L.C., LaMantia A.S., McNamara J.O., Williams S.M., Neuroscience
Robertson D., Biaggioni I., Burnstock G., Low P. A., Paton J.F.R., Primer on the Autonomic Nervous System
Sergeev P.V., Ukhina T.V., Semeĭkin A.V., Akulov Iu.S., Steroid hormones - modulators of the lipid composition of lysosomal membranes of skin fibroblasts.
Tytler C., Historical fact: the Berserkers.
Ueyama T., Emotion, amygdala, and autonomic nervous system