The physiologic modulation of noxious stimuli involves a highly complex system that integrates the actions of multiple opioid receptors and endogenous opioid peptides.
Endogenous opioid peptides
Opioid peptides that are produced in the body include:
- Endorphins
- Enkephalins
- Dynorphins
- Endomorphins
Each family derives from a distinct precursor protein and has a characteristic anatomical distribution. The precursors, prepro-opiomelanocortin (POMC), preproenkephalin and preprodynorphin which are encoded by three corresponding genes code for the endorphins, enkephalins, and dynorphins respectively.
Each precursor is subject to complex cleavages and post-translational modifications resulting in the synthesis of multiple active peptides. The major opioid peptide encoded by pre- proopiomelanocortin is β-endorphin. In addition to β-endorphin, the proopiomelanocortin precursor encodes the nonopioid peptides adrenocorticotropic hormone (ACTH), α-melanocyte-stimulating hormone (α-MSH) and β-lipotropic pituitary hormone (β-LPH). Pre-proenkephalin encodes multiple copies of Met-enkephalin, including two extended forms of Met-enkephalin (a heptapeptide and an octapeptide) and a single copy of Leu-enkephalin. Pre-prodynorphin encodes three opioid peptides of various lengths that all begin with the Leu-enkephalin sequence: dynorphin A, dynorphin B and neoendorphin.The opioid peptides share the common amino-terminal
sequence of Tyr-Gly-Gly-Phe-(Met or Leu), which has been called the opioid motif. This motif is followed by various C-terminal extensions yielding peptides ranging from 5 to 31 residues.
Following the biochemical purification and characterization of the three endogenous opioid peptide families much progress has been made in mapping the specific locations of opioid peptides and their binding site distributions throughout the brain. Pharmacological studies have shown that multiple types (mu, delta, kappa) of opioid receptors exist, and they have indicated that subtypes (mu1, mu2, kappa1, kappa2, delta1, delta2, etc.) are also likely to occur. (See opioid receptors and relative "comments" in this website for more details).
Exogenous application of opiates and opioid peptides has been used to study drug actions down to the molecular level of ion channel modulation and enzymatic regulation. As a model system of putative neurotransmitter peptides, the study of endogenous opioids has the advantage of a relatively extensive list of pharmacologic tools (selective agonists and antagonists) compared to the other peptidergic systems. Despite this significant advantage, two general areas of opioid research have lagged behind. First, receptor purification and cloning has been slow, and results leading to the cDNA and amino acid sequence for the opioid receptors has only recently been obtained, some 18 years after the endogenous ligands were discovered. Second, elucidation of the specific actions of opioid peptides released from endogenous stores has also been only recently described in the central nervous system (CNS). Recent and continuing advances in these general areas should ensure a prominent place for opioids in future neuropharmacological research.
Nowadays we know that:
a) Opioid peptides are synthesized in, and localized to, specific neuronal populations.
b) Enzymatic means for degradation of the peptide has been described.
c) These peptides are released in a calcium-dependent manner from neuronal tissue.
d) Specific binding sites, representing putative opioid receptors, have been localized throughout the brain.
e) Pharmacologic effects have been described in response to exogenous application of the endogenous opioid peptides.
f) These effects can be mimicked by opioid agonists and reversed by antagonists. The principal differences between the actions of opioid transmitters and the more classical transmitters in this region are the relatively slow kinetics of opioid action and the larger radius of action.
Furthermore, our current understanding about endogenous opioids can be summarized as follows:
a) Opioid peptides are stored in dense-core vesicles in specific neurons which also contain a classical fast-acting transmitter such as glutamate; the opioids thus act as co-transmitters serving to modulate the actions of the primary transmitter.
b) The opioids are released into the extracellular space following prolonged depolarization of the neuronal membrane; thus, opioids modulate synaptic transmission only under conditions of intense afferent input.
c) The structure of the opioid peptide synapse may be larger than that of the fast-acting amine transmitters because the peptides may diffuse some distance from their sites of release to their sites of action; this is evident from the slow kinetics of the opioid peptide action (seconds until onset of effects, minutes until termination of the effect). A wider radius of action from the release site follows from the considerably higher affinities the opioids have for their receptors than the amine transmitters have for theirs.
d) Opioids activate a variety of signal transduction processes; different mechanisms are evident in different cell types.
Endorphins
β-endorphin

The term "endorphin" implies a pharmacological activity as opposed to a specific chemical formulation. The term endorphin is a general name for many opioid-like proteins. (It consists of two parts: endo and orphin; these are short forms of the words endogenous metersorphine which means "a morphine-like substance which is produced by the human body").
Endorphins are endogenous opioid polypeptide compounds. They are produced by the
pituitary gland and the hypothalamus in vertebrates during strenuous exercise,excitement, pain and orgasm and they resemble the opiates in their abilities to produce analgesia and a sense of well-being. Endorphins work as "natural pain relievers.” They can be found in more than twenty different parts in the body, such as the pituitary glands as well as in many parts of the brain and nervous system.
Four types of endorphins are created in the human body. They are named alpha (α), beta (β), gamma (γ) and sigma (σ) endorphins. The four types have different numbers and types of amino acids in their molecules; they have between 16 and 31 amino acids in each molecule. Beta-endorphins (β-endorphins) are the most powerful endorphins in the body. They are usually in the hypothalamus and pituitary gland. More endorphins are released in the pituitary gland during times of pain or stress. During severe pain the endorphins in our body cause an analgesic effect to occur, to lessen the pain that is inflicting our body. But during stress, endorphins act differently. They are released in the limbic system which reduces the extent of anxiety that our body is feeling. Not only does the opiate cause the pain to decrease it also causes the feelings of euphoria to occur as well as the release of many sex hormones. Exercise increases the endorphin release too: for the same reason, exercise results in a better mood. Athletes also produce high levels of these peptides. They get a "runner high" when they have done a very hard and strenuous exercise: endorphines allow humans to feel a sense of power and control over themselves in order to persist with activity for an extended time.
Furthermore, endorphins may have a role in preventing obesity, diabetes and psychiatric disorders.
Endorphins act through opiate receptors. β-Endorphin has the highest affinity for the μ1 opioid receptor, slightly lower affinity for the μ2 and δ opioid receptors, and low affinity for the κ1 opioid receptors. μ-Opioid receptors are the main receptor through which morphine acts. In the classical sense, μ opioid receptors are presynaptic, and inhibit neurotransmitter release; through this mechanism, they inhibit the release of the inhibitory neurotransmitter GABA, and disinhibit the dopamine pathways, causing more dopamine to be released. By hijacking this process, exogenous opioids cause inappropriate dopamine release, and lead to aberrant synaptic plasticity, which causes dependency.
Enkephalins
Met enkephalin

Leu enkephalin

Enkephalins are pentapeptides involved in regulating nociception in the body. Discovered in 1975, two forms of enkephalin were revealed, one containing leucine ("leu") and the other containing methionine ("met"). Both are products of the proenkephalin gene.
- Met-enkephalins has Tyr-Gly-Gly-Phe-Met.
Met-enkephalins are endogenous opioid peptide neurotransmitter found naturally in the brains of many animals, including humans.
- Leu-enkephalins has Tyr-Gly-Gly-Phe-Leu.
Leu-enkephalin has agonistic actions at both the μ- and δ-opioid receptors, with significantly greater preference for the latter. It has little to no effect on the κ-opioid receptor.
Dynorphins
Dynorphin A

Dynorphins are a class of opioid peptides that arise from the precursor protein prodynorphin. When prodynorphin is cleaved during processing by proprotein convertase 2 (PC2), multiple active peptides are released: dynorphin A, dynorphin B, and α/β-neo endorphin. Dynorphin A, Dynorphin B contains a high proportion of basic amino acid residues, particularly lysine and arginine as well as many hydrophobic residues and are produced in many different part of the brain. They are stored in large (80-120 nm diameter) dense-core vesicles that are considerably larger than vesicles storing neurotransmitters. These large dense-core vesicles differ from small synaptic vesicles in that a more intense and prolonged stimulus is needed to cause the large vesicles to release their contents into the synaptic cleft. Dense-core vesicle storage is characteristic of opioid peptides storage.
Dynorphins exert their effects primarily through the κ-opioid receptor and act as modulators of pain response, maintain homeostasis through appetite control and circadian rhythm, weight control and regulation of body temperature.
Endomorphins
Endomorphin 1

Endomorphin 2
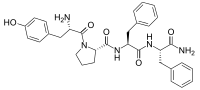
Endomorphins are two endogenous opioid peptides. Endomorphin-1 is located in the nucleus of the solitary tract, the periventricular hypothalamus, and the dorsomedial hypothalamus, where it is found within histaminergic neurons and may regulate sedative and arousal behaviors. It is assumed that endomorphins are the cleavage products of a larger precursor, but this polypeptide or protein has not yet been identified.
They play important role in perception of pain, responses related to stress and complex functions such as reward, arousal and vigilance, as well as autonomic, cognitive, neuroendocrine and limbic homeostasis.
They have the highest known affinity and specificity for the μ opioid receptor.
Endogenous Opioids: Their Physiological Role and Receptors
Neuropharmacology of Endogenous Opioid Peptides
Opioid peptides' different actions at different levels
Molecular level
Opioids primarily act as inhibitory agents in the neural circuit (although examples of excitation are noted below). Opiates and opioid peptides have been shown to inhibit the release of a wide range of neurotransmitters from many brain regions in neurochemical release assays. In the hippocampus, mu agonists have been shown to inhibit the release of norepinephrine and acetylcholine. Kappa agonists have also been shown to inhibit the release of both norepinephrine and acteylcholine. Delta agonists may be effective in inhibiting the release of norepinephrine in guinea pigs.
Cellular level-pharmacologic action
Predominantly inhibitory actions of opioids on neuronal activity and transmitter release are found throughout the CNS. The hippocampal region is one exception to this general finding, because pyramidal neuron excitability is increased following opioid application. It is important to note that no direct effects of opioids on CA1 pyramidal cells themselves have been reported. This paradoxical effect was explained via a mechanism of "disinhibition" in which opioids were acting directly to inhibit inhibitory interneurons, resulting in excitation of the pyramidal neurons. This basic mechanism of opioid action in the hippocampus has been investigated by a number of researchers: recent studies have provided evidence that opioid receptors are specifically present on the terminals of interneurons, acting to inhibit gamma-aminobutyric acid (GABA) release. In addition to disinhibition resulting from the reduction of GABA release, electrophysiological evidence for opioid inhibition of glutamate release has also been recently described.
After having studyed exogenously applied opioids, researchers discovered that the anatomical "mismatch" between localization of opioid peptides and their binding sites is contrary to what would be expected for a classical transmitter such as glutamate, suggesting that they may act in a neurohumoral manner. Much of the added complexity concerning binding site distributions is due to the large species variations; discrete localization of degradative enzymes thought to possibly be involved in the termination and/or spatial restriction of opioid peptide action has also been described.
Enkephalin and dynorphin peptides and peptide-immunoreactivity have been shown to be present in hippocampal tissue: assay methods have been used to measure the relative concentrations present and the amount of peptide released in response to chemically induced depolarization of the tissue. As one would expect for a neurotransmitter, the release of opioid peptides was calcium-dependent. High-frequency trains (>1 Hz) of stimulation were found to be most effective in eliciting opioid peptide release: this may represent a common characteristic of neuropeptide release.

Cellular level-physiologic action
Although granule cells contain the vast majority of dynorphin-immunoreactivity found in the hippocampus, the specific site of dynorphin release was not determined. Release either from recurrent collaterals or from dendrites of the granule cells is possible. Functionally, as the postsynaptic cell is the source of a molecule acting on the presynaptic terminal, dynorphin is acting as a retrograde transmitter. This inhibitory modulation of synaptic transmission mediated by dynorphin may be important in the pathophysiology of the hippocampus, because kappa opioids have been shown to be neuroprotective in excitotoxicity studies. In this role, endogenously released dynorphin could be acting in a compensatory manner to limit hyperexcitability existing during seizure activity. Consistent with this hypothesis is the phenomena of mossy fiber sprouting which occurs in human temporal lobe epilepsies. Contrary to expectations, the time course of this proliferation of mossy fiber recurrent collaterals into the inner molecular layer following a kindling paradigm could not be correlated with an increase in granule cell excitability. Rather, a recovery of inhibition was seen, which is consistent with the inhibitory influence of dynorphin as we have described above. Thus endogenous dynorphin modulation of synaptic activity in the dentate gyrus region may have a prominent role in the pathological and physiological function of the hippocampus.
Numerous studies have described opioid-mediated actions on LTP induction in the two opioid-containing pathways in the hippocampal formation. Although discrepancies among reports have occurred, it can be noted once again that endogenous opioid actions are correlated with high-frequency stimulation events. If one assumes that the phenomena of LTP is representative of learning and/or memory mechanisms, then by analogy with their interactions with LTP, endogenous opioids should potentially affect these processes as well.
Organismal level-physiologic action
Opiate antagonists typically facilitate learning and memory, suggesting that endogenous opioids negatively affect these processes. Unfortunately, most of the studies have involved systemic application of opioids and opiate compounds, rather than local application to specified regions within the CNS. Thus although it is possible to distinguish between peripheral and CNS-mediated effects by utilizing opiate antagonists which do not cross the blood–brain barrier, the specific site of opioid action remains obscure. Studies done testing both peripheral and central administration of agonists and antagonists indicate that peripheral endogenous opioid systems are important in some forms of conditioning.
The hippocampus has been identified as a brain structure necessary for the performance of certain spatial memory tasks. The endogenous opioids do not appear to be implicated in procedural memory (i.e., "learning how" to navigate the maze efficiently), but they do alter declarative memory (i.e., "learning where" the reward is) for a given trial. Given the information concerning opioid peptide localization and release parameters described above, a hypothesis in which dynorphins are released from granule cells following highfrequency stimulation and then act to impair spatial memory is easily supported. This hypothesis gains further support from a study done comparing the spatial memory performance and dynorphin content in young, middle-aged, and aged rats: importantly, animals exhibiting impaired spatial memory performance had significantly higher dynorphin A levels than did their unimpaired, age-matched cohorts. Thus elevated dynorphin predicted impaired spatial memory performance in middle-aged and aged animals, and electrical stimulation of dynorphin-containing cells in young animals also impaired spatial memory.
Opioid peptides play an important role in control of inflammatory pain
Inflammation not only increases expression, transport, and accumulation of peripheral opioid receptors on peripheral terminals of sensory nerves, but also triggers migration of opioid containing immunocytes. Immune cells containing opioid peptides migrate to the inflamed tissue and release endogenous opioid peptides that bind and activate peripheral opioid receptors to produce potent analgesic effect. The degree of endogenous pain inhibition is proportional to the number of opioid-peptide producing cells.
Opioid peptide-containing leukocytes are T and B lymphocytes, granulocytes, and monocytes/macrophages. These leukocytes contribute to endogenous pain inhibition at different stages of inflammation. In early inflammation, granulocytes are the predominant opioid peptide- producing leukocytes, while in later stages opioid peptides are expressed mainly by monocytes or macrophages. Different types of opioid pepetides are released from immune cells: they are derived from distinct genes and respective precursors, which are contained and processed into functionally active opioid peptides by leukocytes.
Opioid containing immunocytes migrate to inflamed tissue in a complex multistep process of recruitment, adhesion, and extravasation controlled by adhesion molecules that are up regulated on vessel endothelia and co-expressed by opioid-containing immunocytes . The adhesion process consists of 3 main steps: tethering, triggering, and latching, and involves sequential interaction with separate classes of adhesion molecules. For instance, selectins mediate the rolling of leucocytes along the endothelial cell wall. Leukocytes are then activated by chemokines released from inflammatory cells and presented on the endothelium. Integrins and adhesion molecules mediate adhesion of leukocytes to endothelial cells and transmigration through the vessel wall into inflamed tissue. Neurokinins (e.g., substance P) contributes to leukocyte recruitment in inflammation by upregulating adhesion molecule expression through neurokinin-1 (NK1) receptors on endothelial cells; augmenting chemokine production; or chemotaxis through NK1receptors on leukocytes. Interference with the recruitment of opioid-containing immune cells into inflamed tissue by blockade of adhesion molecules or by intrathecal morphine injection reduces endogenous analgesia.
Infiltrating lymphocytes express, contain and release opioid peptides within inflamed tissues. Production of opioid peptides in immunocytes is inherently linked with activation of immune cells. Memory type T cells that contain opioids are present within inflamed tissues; naïve cells are not present in inflamed tissue and do not contain opioid peptides. Opioid peptide is released from the immune cells upon stimulation with CRF, noradrenaline, tumor necrosis factor alpha (TNFα), and interleukin 1beta (IL- 1beta), and the corresponding receptors are present on opioid-expressing leukocytes. Multiple areas in the brain, inhibitory interneurons in the spinal cord dorsal horn, and immune cells in subcutaneous tissues, but not the peripheral sensory neurons, co-express CRF receptors and opioid peptides. Adrenergic α1, α2, and β2 receptors are expressed on β-endorphin containing inflammatory cells in close proximity to sympathetic nerve fibers in inflamed paws. The release of opioid peptides is calcium dependent and opioid receptor specific. Secretion requires intracellular calcium mobilization and activation of phosphoinositol-3 kinase and p38 mitogen activated kinase. The immune cells return to the local lymph node after opioid peptide is released and the cells are depleted of the peptide. Consistent with this model, systemic immunosuppression may lead to impaired endogenous analgesia as competent immune cells are essential to achieve release of endogenous opioid peptides within inflamed tissue.
Tissue injury is associated with an inflammatory response, nociceptor activation, and pain. In early stages endogenous hyperaglesic mediators are produced, including cytokines, chemokines, nerve growth factor, bradykinin, prostaglandin, and ATP. Local inflammation also leads to simultaneous secretion of analgesic mediators such as opioid peptides, somatostatin, endocannabinoids,and certain cytokines. The immune system is
a source of opioid peptides and plays an important role in control of inflammatory pain. Inflammatory pain can be effectively controlled by an interaction of opioid receptors on peripheral sensory nerve terminals with opioid peptides released from immune cells upon stressful stimuli. These mechanisms are based on the interaction between the immune and nervous systems.
Peripheral inflammation upregulates opioid receptor mRNA; the synthesis of peripheral opioid receptors in DRG neurons and axonal transport is increased, and there is enhanced G-protein coupling at peripheral nerve terminals in the presence of tissue inflammation.
There appears to be an integral link between infiltrating immune cells and increased presence of opioid receptors on sensory nerves. Inflammation-induced “activation” of the opioid receptors is of significance in the manifestation of peripheral opioid anti nociception; in particular, receptor activation occurs in the early stages of inflammation when the infiltration of thetissue with immune-competent cells is just beginning. In early (within 6 hours) inflammation, leukocyte-derived beta-endorphin, Met-enkephalin, and dynorphin A activate peripheral mu-, delta- and kappa-receptors to inhibit nociception. In addition, central opioid mechanisms seem to contribute significantly to this effect. At later stages (4 days), antinociception is exclusively produced by leukocyte-derived beta endorphin acting at peripheral mu- and delta- receptors. These findings indicate that peripheral opioid mechanisms of pain inhibition gain functional relevance and become more prevalent with duration and severity of inflammation. Corticotropin-releasing hormone (CRH) is an endogenous trigger of these effects at both stages.
The perineurium is a crucial determinant for peripheral opioid analgesia and is impervious in the absence of inflammation. Inflammation increases the permeability of the perineurium, thereby exposing the receptors at the sensory nerve terminals to endogenous and exogenous opioids. Peripheral opioid antinociception and perineural leakage occur simultaneously at a very early stage (within 12 hours) of the inflammatory reaction.
Central inhibition of pain decreases the efficacy of peripheral endogenous opioid analgesia; this was demonstrated by an experimental study in rats. An effective central inhibition of pain apparently signals a reduced need for recruitment of opioid containing immune cells to the injured site.

Neuropharmacology of Endogenous Opioid Peptides
Peripherally acting opioids and clinical implications for pain control