DEFINITION
Pikachurin is a recently identified, highly conserved, extracellular matrix (ECM) like protein with a molecular weight around 110 kDa and high mRNA abundance in retina. It is also known as agrin-like protein (AGRINL) and EGF-like, fibronectin type-III and laminin G-like domain-containing protein ( EGFLAM ). Its gene is localized on chromosome 5p13.2-p13.1 and it is composed of 1017 amino acids.
Pikachurin was first described in 2008 in Japan by the equipe of Shigeru Sato, at the Osaka Bioscience Institute's Department of Developmental Biology, and its name is due to a pokemon, Pikachu, thanks to "lightining-fast moves and shocking electric effects".
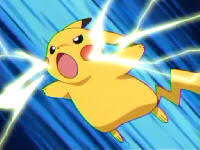
Pikachurin has been reported to bind to α-distroglycan (α-DG) and this interaction has been suggested to contribute to its function in the retina. The full characterization of pikachurin, however, is yet incomplete.
Wikipedia Pikachurin
THE GENE
CHEMICAL STRUCTURE AND IMAGES
Pikachurin's 3D predicted structure:
Front
Top
Side
Pikachurin transcription is regulated by otx2, an important transcription factor for the cell fate determination and development of retinal photoreceptors cells. The gene encodes a 1017 amino acids protein which contains a N-terminal signal sequence, two fibronectin 3 (FN3), three laminin G and two EGF-like domains.
Pikachurin is highly conserved in vertebrates, as indicated by the sequence similarity between mouse and zebrafish in the N-terminal FN3-containing domain (57%) and in the C-terminal laminin G repeats (84%). The C-terminal half of pikachurin showed substantial similarity with agrin and perlecan.
This has been proved by molecular analysys and expression of pikachurin which have demonstrated that the percentage of aminoacid identity and similarity between mouse and human, monkey, cow, chick or zebrafish in each of the amino-terminus portions and carboxyl-terminus is very high.
Pikachurin amino acids percentage:

SYNTHESIS AND TURNOVER
mRNA scondary structure of 3' and 5' UTRs:

Pikachurin-dystroglycan binding is calcium-dependent (it contains different calcium binding domains) and relatively less sensitive to inhibition by heparin and high NaCl concentration, as compared to other dystroglycan ligand proteins. Using deletion constructs of the laminin globular domains in the pikachurin C terminus, Motoi Kanagawa et al. demonstrated that a certain steric structure formed by the second and the third laminin globular domains is necessary for the pikachurin-dystroglycan interaction. In addition, the ability of pikachurin to bind to dystroglycan prepared with glycosylation defects (largemyd and POMGnT1-deficient mice) is severely reduced, suggesting that post-translational modification on O-mannose ( O-mannosyl glycosylation ), which is mediated by largemyd and POMGnT1, is essential for pikachurin binding and proper localization. This suggests that their disruption underlies the molecular pathogenesis of eye abnormalities in a group of muscular dystrophies.
Furthermore, the phenomenon of pikachurin oligomerization, first reported at the ARVO meeting in 2009 and later confirmed by Motoi Kanagawa et al., showed that the first laminin G domain was critical for oligomerization, especially the amino acid sequence from 354 to 498, which includes most of the first laminin G domain. It is likely that oligomerization induces some important conformational change in pikachurin, and a very little oligomerized recombinant pikachurin was found in the conditioned media of Y79 cells.
Post-translational Maturation of Dystroglycan Is Necessary for Pikachurin Binding and Ribbon Synaptic Localization, 2010
Finally, Jianzhong Han et al. showed that pikachurin is post-translationally cleaved in adult retina and human retinoblastoma Y79 cells; this modification is reminiscent of that for dystroglycan. This current study with Y79 cells suggests that endogenous pikachurin mainly underwent cleavage instead of being secreted into the medium as a whole protein.
Cell Specific Post-Translational Processing of Pikachurin, A Protein Involved in Retinal Synaptogenesis, 2012
CELLULAR FUNCTIONS AND TISSUE EXPRESSION
Northern blot analysis of pikachurin transcript in adult mouse tissues:
retina, lung, ovary

After many studies, Shigeru Sato et al. described pikachurin, an extracellular matrix-like retinal protein, and demonstrated his essential role for photoreceptor ribbon synapse formation, apposition of bipolar dendrite and synaptic signal transmission.
Rybbon synapses:
What do they do? | Why are these synapses special? | Why ribbon? | Do they work like conventional synapses? |
They transmit sensory information.The structure and function of this synapse strongly suggest that the ribbon supports continous exocytosis by capturing vescicles from the cytoplasm and transporting them to release sites on the surface membrane. | The electrical signals they transmit are not the same as those in other parts of the brain. In ribbon synapses the amplitude of electrical signals are modified by the intensity of light. | These synapses often have a long, wavy structure which holds synaptic vesicles close to the surface membrane. Sometimes the ribbons tend to be spherical and are also known as "dense bodies"; their shape can change when the neuron becomes more active. | Only ribbon synapses support continous exocytosis regulated by graded voltage signals and tend to contain many more vesicles than conventional terminals. |
Structurally, synapses are specialized sites of cell-cell contact. Cell adhesion molecules and ECM proteins have been suspected, and in some cases have been demonstrated to be important in synapse development and plasticity. Pikachurin seems to be involved in a functional interaction with both dystrophin and dystroglycan at the ribbon synapse.
Pikachurin, a dystroglycan ligand, is essential for photoreceptor ribbon synapse formation, 2008
Interaction and colocalization of pikachurin with dystroglycan (a-f), molecular interaction between pikachurin and α-dystroglycan (g), inhibitory effect of IIH6 on the interaction between pikachurin and α-dystroglycan (h), Pikachurin interaction with eye α-dystroglycan (i):

Furthermore, it has been reported that α-dystroglycan binds to laminins and perlecan in a glycosylation-dependent manner. The inhibitory effects of IIH6 (α-dystroglycan antibody) and divalent cation-dependent binding suggest that pikahurin binds to α-dystroglycan by mechanism similar to those of other known ligands, such as laminins and perlecan. Supporting this idea, pikachurin colocalizes with β-dystroglycan in photoreceptor synaptic terminals. On the basis of these data, pikachurin is likely to functionally interact with dystroglycan components to form proper synaptic connections between photoreceptors and bipolar cells in the retinal ribbon synapse.
In contrast to the postsynaptic localization of dystroglycan in neuromuscular junctions (NMJs), dystroglycan in the ribbon synapse localizes to the presynaptic membrane of photoreceptor synaptic terminals around the bipolar cell dendritic processes. To the best of our knowledge, pikachurin is the first dystroglycan ligand that has been found to interact with the presynaptic dystroglycan.

In addition, Yoshimoro Omori et al. were the first to have discovered that condional deletion of dystroglycan in the photoreceptor presynaptic region causes a defect of pikachurin localization, the prologation of both ERG (electroretinography) amplitude and implicit time (similarly to the deletion of nestin), and e defect of synaptic ultrastructures between photoreceptors and bipolar cells.
A hypothetical model of DG and pikachurin function in photoreceptor synaptic terminals:

Furthermore the loss of pikachurin affects proper dystroglycan assembly in the photoreceptor ribbon synapse and expression of the dystroglycan binding domains of pikachurin in cultured cells causes the clustering of dystroglycan on the cell surface. Especially the clustering of dystroglycan on the cell surface suggests that the interaction between pikachurin and dystroglycan triggers assembly of the dystoglycan–pikachurin complex on the cell surface. Probably, the molecular mechanism for the formation of this complex is that pikachurin oligomerization induces the assembly of the dystroglycan–pikachurin complex. This suggests a molecular mechanism underlying the clustering of the dystroglycan–pikachurin complex in the photoreceptor synapse; both the oligomerization of pikachurin and the interaction of this with dystroglycan cause the clustering of the dystroglycan–pikachurin complex on the cell surface, thus, the dystroglycan–pikachurin complex accumulates on the cell surface. As already seen before, other dystroglycan interacting proteins including agrin, laminin, perlecan and neurexin also contain laminin G/EGF repeats: dystroglycan clustering by dystroglycan-interacting proteins may be regulated by a common molecular mechanism through the laminin G repeat domains. These results strongly support the idea that the interaction between dystroglycan and Pikachurin is essential for the formation of proper synaptic structure of photoreceptors, a “ micromorphogenesis ” of the synaptic terminal. Moreover, this study does not exclude the possibility that the loss of dystroglycan or pikachurin affects the structure and/or the molecular composition also of the postsynapse of bipolar terminals.
Presynaptic dystroglycan-pikachurin complex regulates the proper synaptic connection between retinal photoreceptor and bipolar cells, 2012
DIAGNOSTIC USE
It seems that pikachurin's alterations are related to three diseases:
Unfortunately for retinitis pigmentosa and retinoblastoma studies that relate pikachurin's alterations to their pathogenesis have not yet been conducted. Instead some hypotheses about pikachurin's implications on congenital muscular dystrophies have already been proposed.
Congenital muscular dystrophies (CMD) such as muscle-eye-brain disease caused by defective glycosylation of α-dystroglycan (α-DG) exhibit defective photoreceptor synaptic function. Mouse knockouts of dystroglycan and its extracellular matrix binding partner pikachurin recapitulate this phenotype.
A group of scientists published in 2010 a study named “Pikachurin interaction with dystroglycan is diminished by defective O-mannosyl glycosylation in congenital muscular dystrophy models and rescued by LARGE overexpression”. In this study, they discovered that the expression of pikachurin is abolished at the outer plexiform layer of two mouse models, protein O-mannose N-acetylglucosaminyl transferase 1 (POMGnT1) knockout and Largemyd mice. Overexpressing LARGE restored this interaction in POMGnT1 knockout cells. These results indicate that pikachurin interactions with α-dystroglycan and its localization at the photoreceptor ribbon synapse require normal glycosylation of α-dystroglycan.
The extracellular matrix receptor α-dystroglycan (α-DG) binds to the transmembrane β-DG, which in turn interacts with dystrophin and utrophin via its intracellular domain. Dystroglycan and dystrophin are highly expressed by photoreceptors at presynaptic terminal in the outer plexiform layer. Electroretinography recording of dark adapted Duchenne muscular dystrophy patients with defective dystrophin and the mouse model mdxCv3, a splicing mutation that disrupts expression of the 427 kDa and 70 kDa isoforms of dystrophin, show reduced b-wave amplitude affecting the photoreceptor/bipolar cell transmission. Dystroglycan knockout mice showed similar b-wave abnormalities. These results indicate an essential role of dystroglycan in photoreceptor synaptic function.
Many CMD patients with brain and eye defects have mutations in genes encoding glycosyltransferases (or putative glycosyltransferases) including POMT1 (encoding protein O-mannosyltransferase 1), POMT2, POMGnT1 (encoding protein O-mannose N-acetylglucosaminyl transferase 1), LARGE (encoding like-glycosyltransferase), FKTN (encoding fukutin), and FKRP (encoding fukutin-related protein). POMT1 and POMT2 transfer mannose to serine or threonine residues. POMGnT1 then transfers N-acetylglucosamine to O-linked mannose. LARGE is involved in extending an unidentified phosphoryl glycosylation branch off O-linked mannose and complex N- and mucin-type O-glycosylations of α-DG. α-DG interacts with high affinity to several extracellular matrix components, including laminin, agrin, perlecan, neurexin, and pikachurin. A common molecular phenotype in CMD is the hypoglycosylation of α-DG, which leads to significant loss of its binding activity to laminin and perlecan. Functionally, POMGnT1 knockout mice and the natural LARGE mutant Largemyd mice, exhibit reduced b-waves in their electroretinograms, indicating defective synaptic functions at the photoreceptor synapse when α-DG is hypoglycosylated.
It is not known which extracellular matrix molecule(s) mediates the effect of α-DG hypoglycosylation at the photoreceptor synapse. Pikachurin is a secreted extracellular matrix protein that is expressed at the photoreceptor synapse. Pikachurin knockout in the mouse results in defective ribbon synapses in the outer plexiform layer and a delayed b-wave with reduced amplitude. In this study, they examined pikachurin-dystroglycan interactions in several CMD models. This results show that pikachurin-dystroglycan binding is dependent on proper glycosylation of α-DG, suggesting that defective synaptic functions in CMD may be caused by defective pikachurin-dystroglycan interactions.
Diminished pikachurin expression in the outer plexiform layer of the mutant mouse retinas:

To determine whether α-DG interactions with pikachurin require proper glycosylation, they isolated glycoproteins from whole brain lysates by WGA-affinity from several mouse models,Dag1f/f:Nestin-Cre+, POMGnT1 knockout, and Largemyd mice. Pikachurin overlay and Western blot analysis with IIH6C4 and β-DG antibodies were carried out. In the wildtype, strong pikachurin-binding to α-DG was detected at 125 kDa, while in dystroglycan knockout, pikachurin bound at the 125 kDa location was diminished as expected. A residual signal was detected due to the presence of meninges and blood vessels not expected to express the Cre recombinase in Nestin-Cre transgenic mice. Indeed, remnant amounts of β-DG were detected in the brain lysate of brain-specific knockout mice of dystroglycan. Interestingly, in both POMGnT1 knockout and Largemyd mice models, pikachurin signals at 125 kDa were abolished. In POMGnT1 knockout mouse, weak pikachurin binding at 75 kDa was consistently detected, likely due to the presence of hypoglycosylated α-DG. As expected, immunoblot with IIH6C4, an antibody that recognizes functionally glycosylated form of α-DG, confirmed hypoglycosylation of α-DG in POMGnT1 knockout and Largemyd mice as evidenced by loss of immunoreactivity.
POMGnT1 and LARGE are involved in further extension of O-linked mannose into branched oligosaccharides. Mouse knockout of POMT2 is expected to result in complete absence of all O-mannosyl glycosylation, they therefore analyzed POMT2 knockout neural stem cells. they found no pikachurin binding to glycoproteins isolated from POMT2 knockout cells. Wildtype neural stem cells used as a control showed strong pikachurin binding. IIH6C4 immunoblot confirmed hypoglycosylation of α-DG in POMT2 knockout cells as expected. Taken together, these results indicate that pikachurin-α-DG interactions require O-mannosyl glycosylation of α-DG.
LARGE overexpression enhances pikachurin binding in POMGnT1 knockout cells:

LARGE overexpression hyperglycosylates α-DG and increases laminin binding in not only wildtype cells but also cells isolated from CMD patients with mutations in (presumed) glycosyltransferases. They examined whether overexpressing LARGE had a similar effect on pikachurin interactions with α-DG. In wildtype neural stem cells, overexpression of LARGE increased glycosylation of α-DG as revealed by increased IIH6C4 immunoreactivity with increased molecular weight. Interestingly, pikachurin binding was also increased by LARGE overexpression. In POMGnT1 knockout neural stem cells, we observed only residual pikachurin binding at about 75 kDa. Overexpression of LARGE in POMGnT1 knockout neural stem cells increased not only IIH6C4 immunoreactivity but also pikachurin binding. These results indicate that LARGE overexpression restores pikachurin binding in POMGnT1 knockout cells as well.
In this study, they show that proper glycosylation of α-DG is required for its interaction with pikachurin. Markedly reduced or abolished α-DG binding to pikachurin is observed in POMGnT1 knockout, POMT2 knockout, and Largemyd mouse brains or cells. Furthermore, pikachurin localization at the ribbon synapses was not detected in POMGnT1 and Largemyd mouse retinas. In Dag1f/f:Nestin-Cre+ retinas, ribbon synapses without pikachurin were frequently observed. While manuscript was in preparation, in a very recent study, Kanagawa, et al show that pikachurin interaction with α-DG and its localization at the outer plexiform layer were affected in POMGnT1 knockout and Largemyd mouse retinas. Together, these findings suggest that the synaptic functional defect found in CMD models may be attributable to the diminished pikachurin-α-DG interactions.
Hyperglycosylation by LARGE bypasses deficiency in O-mannosyl glycosylation to rescue laminin binding of α-DG. This unique property has been proposed as a possible therapy for CMD caused by defective glycosylation of α-DG. Their results show that LARGE overexpression also rescues pikachurin-α-DG interactions in POMGnT1-deficient cells, posing the possibility that LARGE may also be used to restore defective retinal functions caused by diminished pikachurin-α-DG binding in CMD. In this regard, it will be necessary to determine which cell type is required to express functionally glycosylated α-DG for maintaining pikachurin expression at the ribbon synapse. The Emx1-Cre knock-in mice used in this study does not drive expression of Cre in the retina precluding cell type specific study with this Cre-driver line. Crossing of POMT2- and Dag1-floxed mice with retinal cell type specific Cre transgenic mice will enable the determination of cell type specific requirement of functionally glycosylated α-DG in pikachurin localization to the ribbon synapses.
POMGnT1 knockout and Largemyd mice exhibit disruptions in the inner limiting membrane. Because pikachurin is not expressed at this location, it probably does not play a role in causing the inner limiting membrane disruptions. Other ligands of α-DG such as laminin-111 may be involved.
Of the extracellular matrix molecules that bind to α-DG, only pikachurin is known to be expressed at the photoreceptor ribbon synapses. No evidence exists that laminin-111, laminin-211, perlecan, and neurexins are expressed at the photoreceptor synapse. However, it is likely some other isoforms of laminins are present in the outer plexiform layer because α3, α4, α5, β2, and γ3 laminin chains are detected by immunofluorescence in the outer plexiform layer. Furthermore, β2 laminin deficient mice exhibit a reduced b-wave amplitude.
Pikachurin interaction with dystroglycan is diminished by defective O-mannosyl glycosylation in congenital muscular dystrophy models and rescued by LARGE overexpression, 2010
AUTHORS:
FRANCESCO SPOLVERATO
FEDERICO GENNARO