DEFINITION
Thyroid hormone 3,5,3′-triiodothyronine (T3) and its precursor thyroxine (T4) are iodinated compounds known to influence gene expression in virtually every vertebrate tissue.
The response results from the interaction of nuclear thyroid hormone receptors (TRs) with specific target gene promoters, a process that can either enhance or repress transcription.
Signalling through this pathway depends on the negative feedback given by changes in serum thyroid hormone concentrations, although it is now known that thyroid hormone signalling can change even as serum hormone concentrations remain normal, thanks to local activation or inactivation of thyroid hormone.

Deiodination is the underlying mechanism of these phenomena.
Deiodinases are the enzymes.
Iodothyronine deiodinase structure and function: from ascidians to humans. 2012
- Abstract
Iodothyronine deiodinases are important mediators of thyroid hormone (TH) action. They are present in tissues throughout the body where they catalyse 3,5,3'-triiodothyronine (T(3)) production and degradation via, respectively, outer (D1, D2)and inner ring (D3) deiodination. Three different types of iodothyronine deiodinases (D1, D2 and D3) have been identified in vertebrates from fish to mammals. They share several common characteristics, including a selenocysteine residue in their catalytic centre, but show also some type-specific differences. These specific characteristics seem very well conserved for D2 and D3, while D1 shows more evolutionary diversity related to its Km, 6-n-propyl-2-thiouracil sensitivity and dependence on dithiothreitol as a cofactor in vitro. The three deiodinase types have an impact on systemic T(3) levels and they all contribute directly or indirectly to intracellular T(3) availability in different tissues. The relative contribution of each of them, however, varies amongst species, developmental stages and tissues. This is especially true for amphibians, where the impact of D1 may be minimal. D2 and D3 expression and activity respond to thyroid status in an opposite and conserved way, while the response of D1 is variable, especially in fish. Recently, a number of deiodinases have been cloned from lower chordates.
In most vertebrates, there are three types of enzymes that can deiodinate thyroid hormones:
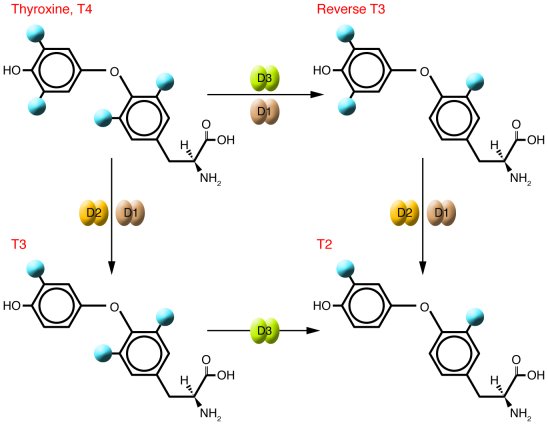
THE GENE
The genes of the deiodinases are expressed in different tissues, as shown in the following picture:

Type II iodothyronine deiodinase is an enzyme that in humans is encoded by the DIO2 gene.
The protein encoded by this gene belongs to the iodothyronine deiodinase family. It activates thyroid hormone by converting the prohormone thyroxine (T4) by outer ring deiodination (ORD) to bioactive 3,3',5-triiodothyronine (T3). It is highly expressed in the thyroid, and may contribute significantly to the relative increase in thyroidal T3 production in patients with Graves' disease and thyroid adenomas. This protein contains selenocysteine (Sec) residues encoded by the UGA codon, which normally signals translation termination. The 3' UTR of Sec-containing genes have a common stem-loop structure, the Sec insertion sequence (SECIS), which is necessary for the recognition of UGA as a Sec codon rather than as a stop signal. Alternative splicing results in multiple transcript variants encoding different isoforms.
CHEMICAL STRUCTURE AND IMAGES
The 3D general model of the deiodinases predicts that the active center is a pocket defined by the β1-α1-β2 motifs of the TRX-fold and the IDUA-like insertion.
The most striking feature of this pocket is the presence of the rare amino acid Selenocysteine (Sec), critical for the deiodination reaction catalyzed by all three deiodinases.
There is sound evidence that all three deiodinases can form homodimers when transiently expressed.
However, it is not clear that dimerization is necessary for function or for other purposes, for example it could reflect associations with other cellular proteins, not primarily involved in their catalytic function, but which could, for example, regulate half-life, transport or subcellular localization.
All are integral membrane proteins of 29-33kDa, and have regions of high homology in the area surrounding the active center.
Based on the HCA analysis, it is clear that the three deiodinases share a common general structure composed of a single trans-membrane segment, which is present in the N-termini of D1, D2 and D3, and several clusters, typical of α-helices or β-strands, corresponding to core secondary structures of the deiodinase globular domains. A striking common feature is the presence of the thioredoxin (TRX) fold, defined by the bab and bba motifs. It is interesting that, within the canonical TRX fold, the relationship between the bab and bba motifs is locally interrupted by interfering elements.
A unique aspect of the deiodinases, however, is that one of these highly conserved intervening elements share striking similarities with a-L-iduronidase (IDUA; 47% identity with D1 and D3, 60% with D2), a lysosomal enzyme that cleaves a-linked iduronic acid residues from the nonreducing end of the glycosaminoglycans, heparan sulfate and dermatan sulfate.

Tertiary structure

The D1 and D2 monomers are Type 1 integral membrane proteins oriented with a small NH2-terminal extension in the endoplasmic reticulum (ER) lumen and a single transmembrane domain exiting the ER at about position 40. This puts the active center of both D1 and D2 in the cytosol.
D3, on the other hand, is a Type 2 integral membrane protein with the opposite orientation of D1 and D2, putting its active center inside the ER lumen (during synthesis) and finally the extracellular space.
Arq Bras Endocrinol Metab vol.48 no.1 São Paulo Feb. 2004
Endocrine Reviews February 1, 2002 vol. 23 no. 1 38-89
Protein Aminoacids Percentage
The three deiodinase proteins (D1, D2 and D3) show considerable similarity (~50% sequence identity).

Protein Aminoacids Percentage
The Protein Aminoacids Percentage gives useful information on the local environment and the metabolic status of the cell (starvation, lack of essential AA, hypoxia)
Protein Aminoacids Percentage (Width 700 px)


IOD1:Responsible for the deiodination of T4 (3,5,3',5'-tetraiodothyronine) into T3 (3,5,3'-triiodothyronine) and of T3 into T2 (3,3'-diiodothyronine). Plays a role in providing a source of plasma T3 by deiodination of T4 in peripheral tissues such as liver and kidney.
IOD2: Responsible for the deiodination of T4 (3,5,3',5'-tetraiodothyronine) into T3 (3,5,3'-triiodothyronine). Essential for providing the brain with appropriate levels of T3 during the critical period of development.
IOD3 :Responsible for the deiodination of T4 (3,5,3',5'-tetraiodothyronine) into RT3 (3,3',5'-triiodothyronine) and of T3 (3,5,3'-triiodothyronine) into T2 (3,3'-diiodothyronine). RT3 and T2 are inactive metabolites. May play a role in preventing premature exposure of developing fetal tissues to adult levels of thyroid hormones. Can regulate circulating fetal thyroid hormone concentrations throughout gestation. Essential role for regulation of thyroid hormone inactivation during embryological development.
SYNTHESIS AND TURNOVER
mRNA synthesis
Endoplasmic reticulum stress decreases intracellular thyroid hormone activation via an eIF2a-mediated decrease in type 2 deiodinase synthesis. 2011
D1, D2, and D3 all contain selenocysteine at their active center, making them part of the 25-member selenoprotein family. UGA encodes for the insertion of selenocysteine during translation, yet in most cases of protein synthesis this codon is normally read as a signal for termination.
Studies revealed the presence of the Sec encoded by UGA, which is recognized in the vast majority of mRNAs as a STOP codon. However, a specific RNA stem-loop immediately downstream of the UGA codon allows for the Sec incorporation in the STOP codon. This structure is termed the Sec Insertion Sequence, or SECIS element, which is present in the three deiodinases and all other selenoproteins.
The SECIS sequence, that binds to its binding protein (SBP-2), which interacts with an elongation factor (EFsec), promotes the insertion of selenocysteine from a specific tRNA (Sec-tRNASec) by the ribosome at the UGA codon.
All the constraints that apply to selenoprotein production will also apply to deiodinase synthesis. This concept has been further validated with the discovery of patients with impaired deiodinase activity due to a mutation in SBP-2.
Since selenocysteine incorporation is not so efficient, several studies have provided insight into the interactions between the components needed to facilitate this incorporation.
A greater affinity for SBP-2 would enable more relative translation of a particular mRNA in conditions of limiting SBP-2. By knocking SBP-2 down in a cell line with endogenous D2 activity, D2 message was unchanged, whereas other selenoprotein mRNA levels decreased.
This suggests that the D2 mRNA has a higher affinity for SBP-2 and is more resistant to nonsense-mediated decay than other selenoprotein mRNAs.
The SECIS element in D2, compared with SECIS element in D1 and D3:

J Biol Chem, Vol. 273, Issue 50, 33374-33378, December 11, 1998
protein synthesis, post-translational modifications
D2 is considered the critical homeostatic T3-generating deiodinase due to its substantial physiological plasticity. A number of transcriptional and posttranscriptional mechanisms have evolved to ensure limited expression and tight control of D2 protein levels, which is critical for its homeostatic function.
D2 activity/mRNA ratios are variable, indicating that there is significant posttranslational regulation of D2 expression.
D2 has a short half-life (∼40 min), it can be further reduced by exposure to physiological concentrations of its substrate, T4, and in experimental situations, rT3 or even high concentrations of T3. This down-regulation of D2 activity by substrate is a rapid and potent regulatory feedback loop that efficiently controls T3 production and intracellular T3 concentration based on availability of T4.
D2 undergoes selective degradation in the proteasome.
Degradation

Selective proteasomal degradation starts by conjugation to ubiquitin (Ub). After Ub is activated by the Ub activating enzyme (E1), the combined actions of Ub conjugation enzymes (E2) and Ub ligases (E3) mediate Ub conjugation to the specific substrate.
Ubiquitinated proteins can then be either degraded in the proteasome complex or deubiquitinated to its unconjugated form.
Studies about D2 ubiquitination were made on cells where E1-mediated activation of Ub can be blocked at high temperatures.
Under these conditions, E1 inactivation decreased the amount of ubiquitinated D2.
T4 can induce D2 ubiquitination even without T3 generation. Importantly, D2 activity is correlated with the amount of D2 and not Ub-D2, indicating that D2 is inactivated upon ubiquitination. In contrast, to this date no ubiquitinated forms of D1 or D3 have been identified under similar conditions, in agreement with the long (>12 h) half-life of these enzymes.
The D2 ubiquitination machinery:

CELLULAR FUNCTIONS
cellular localization

The subcellular location of mature D1 is the plasma membrane.
D2, on the other hand, is an ER-resident protein.
D3 is recycled between plasma membrane and early endosomes. Such recycling probably accounts for the much longer half-life of D3 (12h) than the thyroxine (T4) activating members of the selenodeiodinase family, Type 1 (D1; 8h) or Type 2 (D2; 2h) deiodinase.
Thus, a plasma membrane location for D1 could be viewed teleologically as offering ready access of circulating reverse T3 and T4 to the enzyme as well facilitating the re-entry of the T3 produced from T4 back into the plasma. The localization of D1 in the plasma membrane is in striking contrast to the ER localization of D2.
This differential subcellular localization of D1 and D2 may explain why there is such a minimal contribution of the T3 generated by D1 to the intranuclear T3 in contrast to large fraction of D2-generated T3 to this compartment. The extracellular location of D3 gives ready access to circulating thyroid hormones, explaining its capacity for rapid inactivation of circulating T4 and T3 in patients with hemangiomas and its blockade of the access of maternal thyroid hormones to the human fetus.
localization in tissues
Thyroid hormone signalling in the CNS.


biological function

The activity of the deiodinases can substantially alter thyroid hormone signalling in a given cell.
The Iodothyronine deiodinases are enzymes divided into three types: I, II, and III (D1, D2, and D3, respectively).
They regulate the activity of thyroid hormone by removing specific iodine atoms from the precursor molecule T4.
These three enzymes can activate or inactivate thyroid hormone, depending on whether they act on the phenolic or tyrosil rings of the iodothyronines, respectively.
D2: deiodinates T4, generating the active form of thyroid hormone T3. It is found in heart, skeletal muscle, CNS, fat and thyroid.
D3: inactivates T3 and prevents T4 from being activated. It is highly expressed during development, in fact it is found in the placenta, and then decreases its concentration.
D1: activates or inactivates T4 on an equimolar basis, but is kinetically efficient and of yet unknown utility. It is commonly found in liver and kidney.
Every cell type will express only one type of deiodinase at a given time.
Some tissues express none.
In the pituitary gland there are all three types expressed.
The free concentrations of T4 and T3 are quite similar, even if the total concentration of T4 is twice that of T3, because T4 is tightly bound to TBG (Thyroxine binding protein) and other proteins.
The cytoplasmatic T3 pool includes both T3 from the plasma and T3 generated by D2, alternatively, D3 acts at the plasma membrane to decrease local T3 concentrations.
Thus, the deiodinases are critical determinants of the cytoplasmic T3 pool and therefore modulate nuclear T3 concentration and TR (the receptor) saturation.
Abnormalities in deiodinase activity are important in a number of clinical settings:
Patients being treated with amiodarone, an antiarrhythmic drug, undergo alterations of the thyroid function via both direct actions on the thyroid and inhibition of T4 activation.
Some rare vascular tumors have high D3 activity, which causes severe hypothyroidism in both adults and children.
D3 activity increases dramatically during pregnancy, this may be the cause of increased l-thyroxine requirements in pregnant patients with hypothyroidism. Extrathyroidal pathways have been estimated to contribute about 80% of T3 produced daily in healthy subjects.
The D2 pathway is thought to be the major source of extrathyroidal T3 production in humans.
In contrast, D1 activity is increased in patients with hyperthyroidism so that this pathway becomes the predominant extrathyroidal source of T3.
This D1 predominance in these patients is put in evidence by treating them with hyperthyroidism with propylthiouracil (PTU), an antithyroid drug that selectively inhibits D1-mediated T3 production. Their T3 concentration, suddenly falls down after the treatment.
The D3 pathway is recognized as being the predominant means for clearance of plasma T3: it is clearly shown in patients with the syndrome of consumptive hypothyroidism, in which T4 and T3 clearance rates are greatly accelerated because of the presence of large infantile hepatic hemangiomas with high levels of D3 activity.
A summary of deiodinases' properties is portrayed below:

J Clin Invest. 2006 October 2; 116(10): 2571–2579
Iodothyronine deiodinases: structure-function analysis and their role in the regulation of thyroid hormone levels
REGULATION

The actions of the deiodinases are integrated and thus promote the maintenance of serum T3 concentrations.
Homeostatic changes in the activity of D2 and D3 are regulated by negative feedback:
As the serum T3 concentration increases, D3 is upregulated and D2 is modestly downregulated, decreasing T3 production.
Conversely, if serum T3 concentration falls, D3 would be downregulated, causing the decrease of the clearance of T3.
D1 can deiodinate the phenolic and tyrosil rings of T4 with equal facility, thus its function can be providing some degree of protection against the development of hyperthyroidism when serum T4 concentrations increase, by activating or deactivating T4 when one or the other pathway is necessary.
D2 is also inactivated by binding to ubiquitin, it is then degraded in the proteasome. This ubiquitination accelerates in proportion to T4 concentration, creating a feedback loop controlling D2-mediated T3 production. Therefore, if serum T4 concentrations fall, activation of the D2 pathway compensates by increasing the relative proportion of T4 converted to T3.
The importance of maintaining homeostatic concentrations of T3 is underlined by the fact that mice that had genetic deficiency in the activating deiodinases, had normal serum concentration of T3 and increased concentrations of T4.
The elevations in serum T4 concentration may result from increased thyroidal secretion and/or decreased clearance, but in either case such adjustments in serum T4 concentrations are made in order to maintain serum T3 concentrations.
T3 maintenance can also be pursued by increased thyroid-stimulating hormone (TSH) levels. TSH is released from thyrotroph cells in the anterior pituitary gland, and its role is to stimulate virtually every aspect of thyroid hormone biosynthesis and secretion.
In the absence of TSH, thyroidal activity falls, giving way to secondary hypothyroidism.
TSH release is under negative feedback regulation by thyroid hormone at the pituitary and hypothalamic levels, the latter involving suppression of TSH-releasing hormone (TRH) from paraventricular neurons.
Given that T3 is the active form of thyroid hormone, it is not surprising that a negative feedback relationship exists between plasma T3 and TSH release.
TSH is also regulated by negative feedback by T4, even if this is a prohormone, the fact is that the set point for TSH secretion depends on both serum T3 and T3 generated by D2 in the thyrotrophs, thus TSH rises during iodine deficiency and mild hypothyroidism, situations in which serum T4 concentrations fall before there is any fall in serum T3 concentrations.
Deficiencies in all three deiodinases, caused by lack of selenocystein (an aminoacid needed for their synthesis) lead patients that have this phenotype to have high serum TSH concentrations and serum T4 concentrations.
J Clin Invest. 2006 October 2; 116(10): 2571–2579
Iodothyronine deiodinases: structure-function analysis and their role in the regulation of thyroid hormone levels
DIAGNOSTIC USE
During illness, muscle deiodinase expression changes profoundly, depending on type and severity of illness.
Here is a schematic representation of the alterations in muscle deiodinase expression in mouse models of illness: acute inflammation, chronic inflammation, and severe bacterial infection and sepsis (S.pneumoniae infection, lower panel). On the left side the observed alterations in deiodinase expression, on the right side the theoretical net result on local T3 and T2 concentrations.

Patients with low T3 syndrome have low serum T3 concentrations, which are inversely related to the severity of their illness, and eventually develop low serum T4 and TSH concentrations.
It is believed that decreased extrathyroidal conversion of T4 to T3 is a primary mechanism underlying the low T3 syndrome.
D2 and D3 are primarly involved on the mechanics of low T3 syndrome, but it is thought that T3 and T4 are also directly regulated via a wide variety of intracellular signals not obviously related to thyroid hormones.
While dopamine and glucocorticoids play a suppressive role with respect to TSH, leptin administration leads to increases in serum TSH, T4, and T3 concentrations in fasting rats and humans.
The first evidence of this came from studies in thermogenesis in rats. Cold-induced thermogenesis in brown adipose tissue depends upon the cyclic AMP–mediated acceleration of D2-catalyzed T3 production, which leads to the induction of T3-responsive thermogenic genes.
Without D2 action, brown adipose tissue thermogenesis is impaired, and D2 knock-out mice survive in the cold only by shivering, a behavior not normally seen in small mammals.

In brown adipose tissue, binding of bile acids to a plasma membrane G protein–coupled receptor, triggers an increase in cyclic AMP formation and, subsequently, D2 expression.
In normal mice fed a high-fat diet supplemented with bile acids, oxygen consumption increased and the mice did not gain weight or become as insulin resistant as mice fed the high-fat diet alone.
However, this effect is lost in D2 knock-out mice.
It has been assumed that in humans, the D2 pathway is more important for thermogenesis (mediated by uncoupling proteins) during infancy than in adulthood, based on the fact that infants have far more brown adipose tissue than adults (apart in patients with pheochromocytoma, a tumor secreting catecholamines).
It is now clear that thyroid hormones affect energy expenditure, and that this expenditure is several-fold higher in hyperthyroid as compared with hypothyroid patients.

Furthermore, the thermogenic effect of thyroid hormones is closely linked to increased appetite and lipogenesis to ensure fuel availability and to avoid wasting. Since hyperthyroidism increases hepatic gluconeogenesis and glycogenolysis as well as intestinal
glucose absorption it will exacerbate underlying diabetes mellitus. In lipid metabolism it stimulates lipolysis and there is a cholesterol lowering effect, whereas in hypothyroidism , hypercholesterolemia can be seen.
Neuromuscular effects are described as well, as there is muscle weakness or myopathy, i.e. an increased protein turnover and loss of muscle tissue, and there also is an increase in speed of muscle contraction and relaxation. In children, rapid growth with accelerated bone maturation occurs.
Adiponectin, an adipocyte derived hormone, has been shown to decrease body weight by increasing thermogenesis and lipid oxidation.
Thyroid hormones have similar effect. It was investigated that serum adiponectin level of experimental hyperthyroidism rats was 3.2 fold higher than that of euthyroid ones and had a positive correlation with serum thyroxine.
High serum liver enzyme activities and lower serum cholesterol in cat with hyperthyroidism was observed. In addition hypo- and hyperthyroid conditions had opposing effects on plasma cholesterol levels in mallards. A significant correlation was observed between the cholesterol, triglyceride and the thyroid hormone levels in hyperthyroid patients.
Hypothyroid patients usually had an increase in low density lipoprotein (LDL)-cholesterol. Several mechanisms may have combined to cause a high LDL. For instance, the obese hypothyroid patients had an increase in cholesterol synthesis. Absorption of cholesterol also was frequently increased.
Treatment of hypothyroid patients produced the expected fall in LDL.
One possible mechanism could be that thyroid hormones enhance the conversion of cholesterol into bile acids. However, no evidence was obtained in either hypothyroid or hyperthyroid patients that thyroid hormones alter synthesis of bile acids.
On the other hand, the hormones appeared to increase the synthesis of cholesterol. Patients with hypothyroidism frequently had supersaturated bile. The cause was mostly an enhanced secretion of biliary cholesterol associated with a tendency to obesity and increased synthesis of cholesterol. In contrast, the usually thin hyperthyroid patients did not have supersaturated bile.
The studies show that thyroid hormones a) influence LDL-cholesterol by an action on the catabolism of LDL-independent of alterations in synthesis, catabolism, absorption, or excretion: b) stimulate synthesis of cholesterol ; and c) affect biliary lipid metabolism in large part by influencing energy balance and cholesterol synthesis.
The key observation is that thyroid hormone stimulates expression of the LDL receptor.
T3 thyroid hormone binds to thyroid hormone receptors on the nuclear membrane, the dimer is then imported into the nucleus where it acts as a transcription factor causing, among other effects, LDL receptors to be generated on the cell membrane.
So higher T3 causes the expression of more LDL receptors and that causes more LDL particles pulled into cells and stripped of their fatty cargo. So high T3 tends to reduce serum LDL cholesterol levels, but give cells more energy-providing fats.
Low T3, conversely, would tend to raise serum cholesterol but deprive cells of energy.
The role of deiodinases in that is still uncertain.
From a broad perspective, deiodination of iodothyronines can be seen as an example of a paradigm in which hormones are activated or inactivated in a controlled fashion in specific extraglandular tissues. The deiodinases thus play a role analogous to that of 5α-reductase and P450 aromatase in sex steroid metabolism and of 11β-hydroxysteroid dehydrogenase in glucocorticoid metabolism.
The breadth of actions of these enzymes are only now being recognized; compared with the situation with the steroid metabolizing enzymes, for which multiple antagonist drugs are in widespread clinical use, drug development for the control of deiodination is in its infancy.
The therapeutic potential is both obvious and intriguing: if the D2 pathway can be harnessed pharmacologically, the resulting control of energy expenditure may be useful in the treatment of obesity, type 2 diabetes, and the metabolic syndrome.
J Clin Invest. 2006 October 2; 116(10): 2571–2579
Iodothyronine deiodinases: structure-function analysis and their role in the regulation of thyroid hormone levels
J Lipid Res. 1981
online journal of veterinary research
Iodothyronine deiodinases and cancer. 2011
Thyroid hormones (TH) regulate key cellular processes, including proliferation, differentiation, and apoptosis in virtually all human cells. Disturbances in TH pathway and the resulting deregulation of these processes have been linked with neoplasia. The concentrations of TH in peripheral tissues are regulated via the activity of iodothyronine deiodinases. There are 3 types of these enzymes: type 1 and type 2 deiodinases are involved in TH activation while type 3 deiodinase inactivates TH. Expression and activity of iodothyronine deiodinases are disturbed in different types of neoplasia. According to the limited number of studies in cancer cell lines and mouse models changes in intratumoral and extratumoral T3 concentrations may influence proliferation rate and metastatic progression. Recent findings showing that increased expression of type 3 deiodinases may lead to enhanced tumoral proliferation support the idea that deiodinating enzymes have the potential to influence cancer progression. This review summarizes the observations of impaired expression and activity in different cancer types, published to date, and the mechanisms behind these alterations, including impaired regulation via TH receptors, transforming growth factor-β, and
Thioredoxin and glutaredoxin systems of rat liver cytosol are not influenced by thyroid dysfunction. 1989
The relation of thyroid hormone status to the function of hepatic cytosolic components activating microsomal reverse triiodothyronine (rT3) 5'-monodeiodination was studied in rats. Hyperthyroidism was induced by administration of thyroxine and hypothyroidism, by thyroidectomy. The DTT-stimulated microsomal rT3 5'-monodeiodination rate was increased by 125% in hyperthyroid rats and reduced to about 30% in hypothyroid rats (when compared with euthyroid animals). Thyroid status was unrelated to NADPH-dependent activation of microsomal 5'-deiodinase by cytosol components or to cytosolic concentrations of thioredoxin and glutaredoxin, which stimulate in vitro microsomal deiodination of thyroid hormone.